Flash and JavaScript are required for this feature.
Download the video from iTunes U or the Internet Archive.
Topics covered: Determining hybridization in complex molecules; Thermochemistry and bond energies/bond enthalpies
Instructor: Catherine Drennan, Elizabeth Vogel Taylor
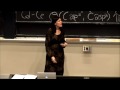
Lecture 16: Thermochemistry
Related Resources
Lecture Notes (PDF)
The following content is provided under a Creative Commons license. Your support will help MIT OpenCourseWare continue to offer high quality educational resources for free. To make a donation or view additional materials from hundreds of MIT courses, visit MIT OpenCourseWare at ocw.mit.edu.
PROFESSOR: OK, let's get started. Everyone can go ahead and take 10 more seconds on today's clicker question. This is about hybridization, which we will go over more to start today, but just to see where we are as a starting point.
All right, great. So most of you got this. The question is what was the hybridization of an atom if we know that it has two hybrid orbitals. This is really, really important, so I'm just going to write this out for that 20% of you that didn't see this right away.
So we know that when we have hybrid orbitals that come from s and p orbitals, these are our atomic orbitals we have to choose from to hybridize. So if we know, for example, that we need two hybrid orbitals, that means we needed to have started with two atomic orbitals. So that means what we would form are two s p hybrid orbitals and we'd be left with our other two p orbitals left over.
So any time you figure out that you're going to need two hybrid orbitals, and we'll go over when you would find this out in just a minute, but any time that happens you know it has to come from an s and a p, so that's why you'll end up with s p hybrid orbitals there.
And exam 2 is happening in just one week from today, so next Wednesday. The semester moves really fast. So I can't believe we're already up to exam 2 talk, but we are. So, you should have all received two handouts when you walked in today, your notes and then an exam 2 info sheet. Did everybody get that? If you didn't get it, just raise your hand and a TA will come to you with that. I see there's a few around, if one of the TA's can jump up and fill those in.
All right, so basically, as with exam 1, all the information you need to know about what's going to be on exam 2 is on this handout. Specifically exam 2 is going to cover lectures 10 through 17. Today is lecture 16, so that means it's going to cover today's lecture and then a bit into Friday's lecture as well, so I'll be really clear on Friday where exam 2 material ends, where you can kind of switch off the exam 2 part of your studying. And it will also be on problem-sets 4 and 5. So it's going to be on two problem-sets instead of three problem-sets, but as you know, there are a lot of different concepts covered in these two problem-sets. So it's still a lot to be thinking about and you need to make sure that you really understand all of these different concepts.
So this is explicitly listed in this sheet here, so make sure you look it over. But I just want to say is well, the concepts that we've covered since exam 1 have been talking about covalent bonds, going along with that are all the Lewis structures that we've done. Then we talked about ionic bonds, so you need to make sure you can solve those ionic bond problems. And then we started talking about different types of bonding in terms of thinking about MO theory, and also vesper theory and hybridization. And then today, and a little bit on Friday, you'll be responsible for some of this new material, which is going to be a little bit of thermochemistry. Not too much on exam 2, most of that will be saved for exam 3 material, but an intro to thermochemistry, that will also be on the exam, just as far as these two lectures.
So, basically this is all written out here, and I also want to mention in terms of your MO diagrams and MO theory, I sent out an email about this, but I also wrote it out here so you have it written down in terms of what you are responsible for in terms of ordering the energy levels of molecular orbitals. So that's all written down explicitly right here, this is what you need to know for the exam. Also it's helpful for the problem-set as well.
And also, in terms of MO diagrams, I think that you will already gone over this pretty explicitly either last week in recitation or yesterday, in terms of what you need to do to get full credit when you're actually drawing an MO diagram. Just in case you don't have that information or you didn't write it down, I wrote this down for you here as well in terms of things like making sure to draw your energy arrows, or remembering to label all of your atomic orbitals. We don't want anyone getting points off on the exam for doing something silly where they do understand what's going on, but they're just not writing it in the correct form.
So all this information is on your sheet here. And also on this sheet mentions that you will be getting optional extra problems on Friday. This is just like for exam 1. The way that you should approach these extra problems, you don't need to turn them in, you're not required to do them, but if you want to do well on the exam we really, really encourage that you do these problems. And in terms of having it be worth your time when you're solving these problems, what you want to do is make sure you're at the point where you can put your notes away, where you can sit alone without your friends, and really approach these extra problems as if they're exam problems. This way if there's some conceptual leap that you haven't quite made yet, but you don't realize it because you've been using your notes or using the conversations with your friends, you want to make that leap and run into those problems alone in your room when you have two hours to figure it out. You don't want to do this leap on an exam.
That's not the ideal situation. So especially with Lewis structures where sometimes you can run into confusions that you need to work through and make sure it all makes sense in terms of your head how to do this, make sure you take the time to do that before the exam and don't have to end up struggling with that on the exam in a time situation.
All right, so the extra problem solutions will be posted on Sunday. And in terms of office hours for next week, my office hours are going to be on Monday from 2 to 4, I'm moving them up, and your TAs will move up their office hours as well, so check with them in recitation or on the website. So that's pretty much it for exam 2. Are there any questions about what you're responsible for or anything regarding exam 2? OK, and in terms of the room it's in Walker again, so at least this part should be familiar. You'll be used to going to your row, it'll be the same row, it's all written here again, but you can just do exactly what you did for exam 1.
All right, so one last class announcement, and this is that if you've been looking at our first sheet of the whole year, sort of the course overview sheet, you know that our class in terms of grading is out of 750 points, and 50 of those points are for attendance and something called occasional in-class quizzes. So what we're going to do is start filling in some of those points because we only have 36 classes worth of attendance. So we have a couple of points to make up in terms of quizzes, and these are going to be clicker quizzes, and let me explain how this is going to work.
Basically, in at least for my part of the class, what we're going to do, because what I really care about is that people are answering the clicker questions, it's really valuable for me. I don't necessarily care if you get them right or wrong during class, because this is the point at which you're still learning them. So these quiz points, which could be up to 2 points per class, will be in addition to attendance points, and you'll get full credit if you answer the quiz question. So you need to be answering the questions because the quizzes will not be announced.
So this is another reward for people that are always participating. These clicker questions are really valuable to us in terms of the feedback, it really gives me an idea of where the class is as a whole. So really, it's important that you are answering these questions and this is a way to get your quiz points up, so in addition to attendance, you'll get 2 quiz points. We'll have our first unannounced quiz today, so you won't know what problem it is beforehand, so I suggest you make sure you're answering all the clicker questions, which most of you do anyway. And I will just say I recognize that some of you are just scurrying across campus to get here on time. So I'll never make that first question a quiz question, because I know how hard it is to get from one place to another on this campus, and I know most of you do a great job of sneaking in right at the last minute when you have that long run. So we won't make the first question a quiz question, but be on the look out for a quiz question today.
All right. So let's get to today's topic. We're going to finish talking about valence bond theory and hybridization. So what we've already done is really cover all the theory that we're going to cover behind how hybridization works, and behind how valence bond theory works. But what we haven't covered is how do we actually solve problems doing this in the really quick way. So we're going to do that. This is going to be a more practical lesson in hybridization. And once we do that, we'll move on to now talking about energies and enthalpies of chemical reactions.
So this is a real shift. We spent a while talking about single atoms, and then we've spent most of the material, since exam 1, talking about bonding. So now we're taking it one step further and we're going to actually get to talk about some chemical reactions. So we'll do that once we finish up with our hybridization unit here.
All right, so how do we go about determining hybridization in complex molecules? It's actually incredibly simple, and all that you need to do is think about for the given atom that you're looking at, what you want to do is add up the number of atoms that are bonded to your central atom, or the atom you're considering, and add to that the number of lone pairs. And what you end up with is the number of hybrid orbitals that you need.
All right, so the theory behind hybridization and picturing everything, that can be pretty complicated, and I do want you to understand that. But if, for example, on an exam situation, you don't necessarily have to think through everything, you can just use this very quick way to figure out the number of hybrid orbitals that you need. And what we just said is that any time we need two hybrid orbitals, we know that the hybridization of that atom is going to be s p, because it's made up of one s and one p orbital. So let's say we need three hybrid orbitals. What is our hybridization of our atom in this case? Yeah, it's s p 2. We want to take three atomic orbitals to make three hybrid orbitals, so it's going to be s p 2. So what if we need four hybrid orbitals, what's the hybridization going to be in this case?
STUDENT: S p 3.
PROFESSOR: S p 3. All right, great. So, really that's it. Hopefully hybridization just got a lot simpler for any of you that have been struggling a little bit with it. But let's go ahead and do an example to make sure that we can all do this. And let's take a look -- first let me mention an exception here. The one exception to thinking about how many hybrid orbitals that you have are in the case where you have single bonded terminal atoms. So in the case of a terminal atom that is a single bond, you're not going to hybridize it. So in that case, just don't even change anything it's just going to be one of the p orbitals, or unless you're talking about hydrogen in which case will be an s orbital that overlaps.
All right, so we can illustrate this with an example of formal chloride. So this is a good example because we're just dealing with our carbon as our central atom here, and we have three terminal atoms, two of which are single bonded, so we're not going to hybridize those, and one which is double bonded, the oxygen, so we will hybridize that one.
So in terms of thinking about the carbon, what is a hybridization around the carbon atom?
STUDENT: [INAUDIBLE]
PROFESSOR: All right, I'm hearing some mixed answers. So let's think. So the carbon atom is bonded to three different atoms, and it has no lone pairs. So what's the hybridization?
STUDENT: S p 2.
PROFESSOR: Good s p 2. So, if we're talking about the c h bond, we're going to say that it's carbon, it's a sigma bond because it's a single bond, and then it's carbon 2 s p 2 bonded to a hydrogen 1 s orbital. All right, so let's take a look at the carbon chlorine bond here. Again, carbon is still 2 s p 2, it's the same carbon atom, and it's going to be a sigma bond because it's a single bond, but what about the chlorine, what atomic orbital is going to be bonding in the chlorine?
STUDENT: [INAUDIBLE]
PROFESSOR: All right. I'm hearing a little bit of mixes, they're all p's, which is a good start. It turns out that it's going to be the chlorine 3 p z. So the reason that it's p is because we're not hybridizing it, and the reason that I specifically say that it's the p z instead of the p x or the p y, is remember that the z-axis, that's our bonding axis, that's our internuclear axis. So any time we have a p orbital that's involved in a sigma bond, it has to be the z orbital because that's the only one that has the right orientation to overlap along that z-axis. So we're going to say it's carbon s p 2, and then chlorine 3 p z.
All right, so let's look at the last bond here, which is a carbon oxygen double bond. We know that any time we have a double bond it's made up of one sigma bond plus one pi bond. So, when we look at the carbon again, that's 2 s p 2. But what about the oxygen, what's the hybridization of this oxygen atom? S p 2. So we're going to have carbon 2 s p 2, and then oxygen 2 s p 2. It's s p 2 because the oxygen is bonded to one atom plus two lone pairs, so we're going to have a total of three hybrid orbitals.
All right, so this is not our only bond, we have a double bond, so we also need to talk about the pi bond. So if we talk about the pi bond, we can say that's carbon 2 p y, then the oxygen 2 p y. We could alternatively say, and be correct, that it was the carbon 2 p x and the oxygen 2 p x. Either one of those is fine.
All right, so that's an example of how we can assign very quickly and very easily what the hybridization is of a given atom. And then also fully describe the symmetry and the atomic or hybrid orbitals that make up bonds. So this is a lot of your problems on the p-set, so if you haven't finished that section, hopefully you'll be able to get through that section pretty quickly when you go back to it.
And let's take a look at a little bit of a more complex molecule here, which is ascorbic acid or vitamin C. And this is a good example because it's starting to look a little more complicated, but not too much more complicated. But the reality is if you know how to do assigning the hybridization this way, even if I gave you a 1,000 atom protein, you should still be able to get it completely correct.
Vitamin C is a really important molecule to actually think about in terms of thinking about its shape and its hybridization. Vitamin C is an antoxidant. So we might remember that from when we were talking about free radicals. Free radicals can cause oxidative damage. These antioxidants, some of our vitamins, including vitamin C are antioxidants. So that's something that a lot of people in the general population know if they're into their health.
But another really important thing about ascorbic acid is that it's an enzyme cofactor. And a cofactor just means that it's some type of molecule or atom, in this case it's a molecule, that's required by an enzyme in order for the enzyme to carry out its chemistry. And the chemistry that the enzymes that vitamin C are a cofactor for are responsible for putting oxygen, o h groups, onto collagen molecules in order to form what is the collagen triple helix. And collagen is the main constituent of bones, of joints, of connective tissue, of many important structural parts of our bodies. So you can imagine that vitamin C is very important because we need to keep our collagen intact. Collogen is actually one of the most prevalent proteins in our entire body, and it makes up a large part of our cells. So you can imagine if we don't have collagen that is in this triple helix, we're going to run into some problems. Does anyone, or I'm sure many of you do know, but can someone tell me what the name is of the disease if you have a deficiency in vitamin C?
STUDENT: Scurvy.
PROFESSOR: Yeah, scurvy. So scurvy, most often associated with ships because of the 1500's and 1600's and 1700's when boating technology was far above biochemistry technology, so people could make ships and go on these long journeys, but people did not yet understand they needed to keep certain vitamins in their bodies, such as vitamin C. Maybe also, they didn't know about -- well they didn't even have the structure, so they couldn't have realized that vitamin C is polar and won't stay in their bodies for very long, it will get excreted out through their urine. So they were not thinking about how quickly they could run into trouble in the long sea voyages.
And I don't know how many of you had a unit on explorers in elementary school -- I did in fifth grade, and one that always sticks in my head is Magellan's trip around the world, who led the first fleet of ships around the globe. What they did not mention to us in fifth grade was that 90% of his men died on that journey. And I understand we were young kids, I didn't want to know that at the time, but it's interesting and it's really important, and the reason for many of these deaths was scurvy. And it's totally preventable. And, in fact, the cure for scurvy, which is as simple as taking vitamin C in any form, was known for -- since the fifth century people started figuring this out, but the problem was there was so much false information as well that it was not a general practice on ships to be treating men that were suffering with vitamin C or using it as any kind of preventative measure.
The other somewhat interesting thing I want to say about vitamin C is that it was part of the first ever published clinical study. And this was a controlled clinical study that was done in the 1700's, the first one that's ever been reported, and not surprisingly it was done on a ship and it was done by James Lind who was a Scottish naval surgeon. And basically, he took 12 of his men, so this was not a very large clinical study like they do today, but he took 12 men that were suffering from scurvy, and he gave them a diet mostly of just carbohydrate mush, because when you have scurvy, one of the first signs is bleeding gums and teeth falling out, they couldn't really eat too much. But he supplemented their diet. He put them in pairs of two and supplemented with 1 of 6 things. So two of them got a pint of cider a day to see if that helped. Two of them got half a pint of seawater -- maybe not the best group to be in. Even worse group to be in is two of them got diluted sulfuric acid. Tuned out that didn't help so well. Other things where some got spices, not so bad. And then two of them actually got citrus fruit. So no big surprise ending to this clinical study, the people with citrus fruit were completely cured and back to duty within a week, and I'm not sure exactly what happened to everyone else, but let's hope they got switched over as well.
But it's just neat to think about even back then, people were doing these controlled scientific studies, this was one of the first cases. Unfortunately, it was another 40 years between this study being published and the British Navy requiring supplements of citric acid. So, really, we've come a long way in terms of disseminating scientific knowledge to the community, and that's a really important part of being scientists -- not just making these discoveries, but also passing along, for example, to these ships full of people that need to know it.
So, in terms of scurvy, we don't see too much of it today, but who should be concerned about suffering from scurvy? Do we have to worry about our cats or dogs at home, are they getting enough of their vegetables and vitamin C? It turns out we don't have to worry. Primates are who should be concerned. So we need to be concerned, other primates need to be concerned. It turns out that most other mammals actually biosynthesize vitamin C, so we don't have to worry about most of our pets at home. Unless you have a guinea pig and they don't, in fact, biosynthesize vitamin C, so I think they supplement most guinea pig pellets with vitamin C, but maybe keep an eye on your guinea pig. We primates and guinea pigs are the only types of mammals that don't actually biosynthesize their vitamin C.
So again, vitamin C is a cofactor, that means it needs to bind to an enzyme, it means that the shape of vitamin C and the hybridization are going to be really important. So let's take a look and talk about some of these carbon atoms here. And you can look at your structure of vitamin C in your class notes while you're doing this, and first I want you to tell me what the hybridization is of that carbon a in the vitamin C molecule. And let's take 10 more seconds on that.
OK, 78%. We'll do another question like this in a minute. So if we can switch back to the notes we'll take a look at that. I think we can get better, I think we can get to the 90's with these hybridization, we just need to follow the rules. So if we're thinking about vitamin C and we're talking about carbon a, how many things is carbon a bonded to?
STUDENT: [INAUDIBLE]
PROFESSOR: four things. We have two hydrogen atoms, an oxygen, and then another carbon. So that means we need, if it's bonded to four things, we need four different hybrid orbitals, so it needs to be s p 3 hybridized. All right, let's try this again but just shouting out, what is the hybridization of carbon b? Good. OK, I'm going to say that was about 90% of you I heard, which is excellent. S p 3. What about carbon c here? Yup, s p 3 again. Bonded to four things it's s p 3. What about carbon d? S p 2, great. Carbon e?
STUDENT: S p 2.
PROFESSOR: All right, and this last one, the last 10% of you can join in. Carbon f? Great, s p 2. All right, so we can also think about shape once we know hybridization and how many atoms a carbon atom is bonded to. What would we say that the shape is of these s p 3 orbitals -- or the shape of the carbon atom. Yeah, it's tetrahedral. So this is going to be tetrahedral. And what about carbon d, e, and f? What is the geometry of carbon d, e, and f? Good, trigonal planar.
All right. So let's actually take this one step further from just talking about the hybridization of the individual atom. Let's talk about a couple of these bonds. I won't go through all of them. I think you can get the idea with just a few, but you can go back and try all of them later.
So let's start with thinking about carbon b hydrogen bond. Is this going to be a sigma or a pi bond? Sigma. We know it's sigma, because any time we have a single bond it's a sigma bond.
So carbon b, we just said, is carbon, it's going to be 2 s p 3. And then hydrogen, what's the atomic orbital we're talking about here? 1 s. All right, great. So let's take a look at another one, let's look again at carbon b, but now let's talk about it bonding to its oxygen there. So again, are we going to be talking about a sigma or a pi bond? A sigma bond, because again, it's a single bond here.
So what we'll say again is carbon 2 s p 3, and let's take a look at this oxygen that it's bound to. Is this oxygen -- what is the hybridization of this oxygen right here? What is it? OK, good, it's s p 3. It's bound to two atoms, plus it has two lone pairs, that's a total of four things. So it needs to have three different hybrid orbitals, so we'll say it's oxygen 2 s p 3.
So let's skip to a different carbon now, let's talk about carbon d, specifically the carbon d oxygen bond. So let's do a clicker question for this one. Let's get into the 90's, if you don't mind, this would be very good to do. It'll make me feel better about you finishing your problem-sets tonight. So if you talk about the symmetry and the hybrid or atomic orbitals that contribute, we're talking about the c d oxygen bond. So go ahead and look at your notes and see what that bond is. We already identified the hybridization of carbon d, so we really just need to think about the oxygen here. All right, let's take 10 more seconds.
OK, I'll take that, 84%. That's not bad. So again, we're looking at a sigma bond here. It looks like the one people got it confused with was talking about oxygen s p 2 versus s p 3. Remember, you just need to look at what it is actually bound to, so for carbon d, the oxygen's bound to two atoms plus two lone pairs, so it's going to be s p 3 hybridized. So we'll call this a sigma bond where we have carbon 2 s p, now it's s p 2, and oxygen 2 s p 3.
All right, let's take a look at just one more here. So, let's look at the carbon d bond with carbon e. So if we can switch back to the class notes just to see that, though you guys can see it on your notes as well. So in terms of that, we're talking about a double bond now, so we know that we have to have one sigma bond and one pi bond to completely describe our double bond. So for the sigma bond, again, it's going to be carbon 2 s p 2. And now we're talking about the other carbon. What is the hybridization of the other carbon? Yup, s p 2. So carbon 2 s p 2. All right, but we're not done there. We also need to talk about the pi bond. So for the pi bond, we'll talk about carbon 2 p y, and then the second carbon 2 p y.
All right, so I filled in a few more in your notes, so you can maybe cover those up and make sure that you get all of those correct is a good self test before you finish off any in your problem-set. Does anyone have any questions about figuring out hybridization? Yes?
STUDENT: [INAUDIBLE]
PROFESSOR: I'm sorry, what is that? In this bond here? Oh, OK, that's a good question. So the question was why is there not hybridization in these p orbitals here. So in order to form a double bond, we need to have a pi bond forms, and a pi bond forms from two unhybridized p orbitals. And if you can kind of picture those p orbitals, if our molecules are this way and they're coming up here, we need to have electron density above the bond and below the bond. So if they're hybrid, then they're going to be spread out into that tetrahedral geometry. We want to have them parallel to each other and they need to be the p bonds, they need to be the p orbitals. Does that makes sense? So any time we have a double bond, the pi part of the bond is going to be p orbitals. Yes?
STUDENT: [INAUDIBLE]
PROFESSOR: No, absolutely not. It would be absolutely correct to put 2 p x and 2 p x here, you just can't put z, because that's the one that's going to be involved in the sigma bond. And the other important thing is if you do put x for one, you have to make sure you put x in the other, because they do need to be able to interact with each other. You can't have one x and one y, but sure, you can have both x or both y.
OK, so let's shift gears a little bit and start talking about bonding in terms of chemical reactions. So we're going to start today with talking about bond energies, and also something called bond enthalpies. And the first point is just to bring us back to something we're very familiar with. When we talked about covalent bonding, a concept that we have been discussing is bond dissociation energy. That's just the energy that's required to put into a molecule in order to break that bond. This is something that we saw when we were talking just at the very beginning of our unit on covalent bonds.
That energy difference is the energy difference between when we have the c h 4, if we're talking about methane, versus one of those hydrogen bonds breaking, one of those c h bonds breaking. So we've talked in the past about bond energy, but what I want to introduce to you today is a very related concept, which is called bond enthalpy. So that's delta h here is what we call bond enthalpy.
So, this is talking about instead of the change of energy accompanied with a bond breaking, we're talking about a change in heat accompanied with a bond breaking. So whether when that bond breaks it requires heat, or whether it gives off heat when you break that bond or talking about a reaction. That's what we're talking about when we're talking about enthalpy. It turns out that enthalpy is, in fact, very related to energy, and we can relate it with this equation here that bond enthalpy is equal to bond energy plus the change in pressure times volume.
So we could, in fact, go back and forth between bond energies and bond enthalpies, but the reality is if we're talking about gases, which we are in many cases, then what we find is the difference between bond enthalpy and bond energy is only 1% to 2%. So it's not very significant. And, in fact, if we're talking about solids or liquids, now we can say that the difference between bond energy and bond enthalpy is going to be negligible.
So, for the rest of this class today, we're going to stop talking about bond energy, which I did just for a moment, to orient you back to a discussion that was familiar, we're going to switch our discussion to bond enthalpies. One reason that we like to talk about enthalpies is unlike energy, which can be a little bit more tricky, bond enthalpies are easy to measure. It's easy to measure how much heat a reaction gives off or how much heat a reaction takes in.
So we can also talk about something, which is called the standard bond enthalpy, so any time you see this symbol here, which looks like a knot, so it looks like a delta h knot, that refers to the standard bond enthalpy. Any time we we're talking about a standard, whether it's enthalpy or, as we'll see in the next lecture, standard entropy or a standard free energy, we're just saying that the molecules that we're talking about are in their standard states, which means they're in their pure form. If they're a gas it means they're at one bar. Usually this is referring to room temperature, so 298 kelvin. It's often that when you look up tables of different bond enthalpies, you'll see them in their standard state and it will be at room temperature.
So if we talk about standard bond enthalpy, let's talk about what this is for these c h bonds, which we just saw in methane. So if we're going from c h 4 to breaking one of these c h bonds, what we see is that the bond enthalpy is 438 kilojoules per mole. The fact that it's positive tells us that we need to put in that much heat into the system in order to break that bond.
So we now know the enthalpy of a c h bond, but, of course, methane is not the only kind of c h bond that you can envision. We could talk about the c h bond, for example, in ethane or trifluoromethane or trichloro or tribromomethane, and what you'll find is that the amount of enthalpy that that reaction is associated with depends on the exact type of c h bond that you have. So, for example, we can see that we have slightly different, not vastly different, but slightly different changes in enthalpy depending on which kind of c h bond that were breaking.
And what I want to point out also is that all of these delta h's, all of these standard bond enthalpies are positive. Any time we have a positive delta h, we call this an endothermic reaction. And again, endothermic just means that the reaction takes in energy. It's very similar to what when we're talking about energy whether something's taken in or released, whether it's positive or negative. If you have positive delta h, it's endothermic, it takes in heat in order for the reaction to go.
So we can think about instead of talking about all of these individual c h bonds, instead we can talk about what the average value would be for all c h bonds. So we can say that the average of any c h bond is 412 kilojoules per mole. So you can imagine if you're looking these things up in terms of a reference table or maybe in the appendix of your textbook, it would be an impossibly huge number of pages to write every single different kinds of c h bond. So that's why instead they talk about the average bond enthalpies. So for c h, again, that's 412, and all of the enthalpies we just saw fall within about 8% of that. And in your book you can look up any type of bond that's listed here. So we saw c h is 412. You can look up a c c bond, you can look up a c c double bond, a c c triple bond, and so on. And you'll note that what these are are mean bond enthalpies, so they're the average of all different types of c h bonds or c c bonds that you can imagine.
So, you might be asking what's so important about being able to look up and think about these different bond enthalpies. And the reason that it's important is because if you're looking at a reaction, no matter how complicated that reaction is, you can actually figure out what the enthalpy of the entire reaction is by adding up all the individual mean bond enthalpies of the products, and all the individual mean bond enthalpies of their reactants and thinking about the difference between those two. So we'll do that in just a second, and the reaction that we'll do it with is the oxidation of glucose here. So, c 6 h 12 o 6, one mole of glucose plus six moles of oxygen, gives us six moles of carbon dioxide and six moles of water.
So what we can find out, and hopefully what we will match up when we look at using the different bond enthalpies, is that the enthalpy of this entire reaction is negative 2816 kilojoules per mole. So in this case we're saying that delta h is negative. Does anyone know what it's called when delta h is negative? Everyone knows, great. So it's exothermic. This is an exothermic reaction, the reaction releases heat.
So I just want to mention before we go on, if you look at almost any freshman chemistry textbook, what you'll find is this oxidation of glucose reaction is used a lot in talking about thermochemistry. And one reason it's talked about is because it's very convenient to talk about something where we start with one mole of glucose and end up with 12 moles of products. That's going to be helpful what we're doing a practice problem to see exactly how you deal with it when there's different numbers of moles.
But the other reason you always see this reaction is because it's an incredibly important reaction. The oxidation of glucose is going on all the time in our body, this is our main source of energy for all animals. So let's think a little bit about why this reaction is so important and so prevalent. It turns out that if we're talking about plants, plants do the reverse reaction. So plants take carbon dioxide and water and they turn it into glucose or energy for us, energy stored in the bonds of glucose plus oxygen. So if we do the reverse reaction, this is actually going to require energy. Where do plants get this energy? Yeah, this is just photosynthesis here. This is the photosynthesis where plants are turning carbon dioxide and water into sugar and into oxygen.
So what happens when we eat the plants or when we eat animals that have eaten the plants is that we perform the reverse reaction now, which is what I just showed you, the oxidation of glucose. And even though it's not the products of the reaction that are particularly valuable to us, we just breathe out the c o 2, and actually we'd rather have less of that than more in our environment, but what's important here is instead the energy or the enthalpy that's given often in this reaction. So as I said, this reaction has a negative enthalpy of 2816 kilojoules per mole. That's a lot of enthalpy and a lot of energy. So we actually end up using that energy to fuel most of what is going on in our bodies. So instead of storing it as sugar, once we oxidize the sugar, now we just store it as ATP, and as you know, ATP is the currency of energy in the cells.
So this is why you see this reaction again and again and again in just about any chemistry textbook that you open up as sort of a general reaction. The reason it's used is it's just so important and so prevalent in terms of thinking about our bodies and how we're staying alive and using energy.
All right, so let's go ahead and use this as an example of what we just said, which is using the bond enthalpies of the products and the reactants to figure out the enthalpy of the entire reaction. So the way that we do this is we add up all of the individual mean bond enthalpies of the reactants, and we subtract from that all of the individual bond enthalpies of the products. So we can think about what this will tell us. If you think about the fact if the bonds are stronger in the products than they were in the reactants, you can go ahead and click in and tell me if you think we'll have a negative or a positive delta h here.
All right, let's take 10 more seconds on this. OK, great, so negative is correct and some people got totally mixed up. Didn't just get one thing -- so let's just focus on the right answer to start with. The correct answer is that it's negative, it's an exothermic reaction. And actually, lets switch to our class notes to explain why. And also, this was the quiz question for today, so whether you got the answer correct or incorrect, you get full quiz points if you did, in fact, answer. But let's still focus on the right answer here and see why it's correct.
So if we're talking about the bonds being stronger in the products, that basically means that we ended up releasing a lot of heat when we made those bonds and the products, and we didn't have to use up too much of that heat to break all the bonds and the reactants. So that's why we say that delta h is going to be negative. You could also just do it not conceptually, just plugging it into the equation, but it's better to kind of understand exactly why that is. It's because it takes more energy -- you gain more energy forming the products than you take breaking up the reactants.
So if we have the opposite case here, if the bonds are stronger in the reactants, now what we're going to find is that the delta h of the reaction is positive and what you're dealing with is an endothermic reaction.
So let's go ahead and do this with our example of the oxidation of glucose. So, I've just written out the glucose molecule so you can see all of its individual bonds here since we're going to be using that information. So let's start by talking about the bonds that are broken in terms of thinking about all of the reactants here. So if we're talking about the sugar molecule itself, what we have is we have seven c h bonds. And if we add up all the o h bonds, we have five of those. How many c o single bonds do we have? What do people think? Yeah. five c o single bonds. What about c c single bonds? five of those as well. C o double bonds? Just one c o double bond. And then we have our oxygen molecule to worry about and we have six o o double bonds.
So if we add up all of the bonds are broken, and we subtract from that the bonds that are formed, those strengths, the c double bond o, we have 12 of those. And how many o h bonds do we have? Right, 12 as well. So if we add up all the bonds broken, what we end up with is 12,452 kilojoules per mole, and it's talking about per mole of glucose that's oxidized. And in terms of bonds formed, what we see is 15,192 kilojoules per mole.
So all we need to do to figure out the change in enthalpy, and when it has this subscript r here, I meant to mention, that means the enthalpy for the reaction. We just subtract these bonds here from the ones that we ended up forming. So basically, what we're saying -- excuse me, these are the ones that we broke. It's 12,452 minus 15,192, which we formed. So we end up with an enthalpy of reaction of negative 2,740 kilojoules per mole of glucose that's oxidized.
All right, so if you remember the number that I told you before, it doesn't exactly match up with what we had said is the exact number. The exact number is 2,816. It is within 3% though, that's pretty good. Because remember, we're not using exact bond enthalpies here, what we were using is average bond enthalpies. So it makes sense that we're going to be a little bit off. But if all you have in front of you is the information on bond enthalpies, mean bond enthalpies, this is a great way to figure out the enthalpy of an overall reaction.
We can think of a different way, however, to think about the enthalpy of an overall reaction, and this is talking about the heat of formation. And the heat of formation or delta h formation here is equal to the heat of -- or the enthalpy of reaction, if we're talking about forming one mole of compound from its pure elements, which are in their standard states. So basically, there's a table that you can look up which tells you the enthalpy of formation for any compound that you're interested in, and this is actually an appendix to of your textbooks, and you will need to use this to solve your problems on p-set. But let me say that, for example, if we're talking about water here, that's formed from hydrogen and oxygen, if we're talking about the elements in their pure forms at one bar, standard states, and room temperature. And if we look this up in our textbook, we would find that the delta h of formation for water is negative 286 kilojoules per mole.
Similarly we can look up the same thing, for example, for carbon dioxide, and what we'll find here is that our delta h of formation that we look up is negative 393 . 5 kilojoules per mole. We can also look up or think about what our delta h of formation would be for oxygen. Does anyone have a guess here? Yup, it's actually going to be zero. Oxygen already is in its most stable state, so any time we're talking about an element in their most stable state, it's going to be zero. That's going to be the delta h of formation, there is none, it's zero.
We can also look up what it was for sugar, for glucose, c 6 h 12 o 6. So that coming from its most stable forms, it was the most pure form of the elements, is going to be negative 1260 kilojoules per mole. All of this information has been tabulated and is in tables, you can refer to them, and they're also in the back of your textbook, so you can refer to those in terms of your problem-set.
So let's go ahead and solve a problem actually using the delta h's of formation. Let's see if we can do any better to coming close to the reality for the oxidation of glucose. So if we're going to calculate the delta h for the reaction for the oxidation of glucose, or actually for any reaction at all, what we want to do is take the delta h of formation of the products and subtract from that the delta h of formation of the reactants. So let's go ahead and do that. And in terms of talking about the oxidation of glucose, if we talk about delta h of this reaction, what we need to take is 6 times delta h of formation of c o 2, since we're forming, that's in our products, we're forming six moles of c o 2. Plus 6 times delta h formation of h 2 o, since we're forming six moles of h 2 o. And we subtract from that the heat of formation of all of our reactants.
So we only have one mole, so we just say delta h formation of c 6 h 12 o 6, and then in addition to that we have six moles delta h formation of oxygen, of o 2.
All right, so this is our equation here and at this point what we would do is we would look up what all of the delta h formation values are for c o 2, h 2 o, sugar, and oxygen. So what we would find is that we end up having 6 times negative 393 . 5 -- that's what we had just looked up and told you for c o 2. Plus 6 times negative 285 . 8 for the water. Minus 1 times 1260, so it's negative 1260 for our sugar here. And then what we're going to end up with is having minus, and what is it for oxygen again? So minus 0.
So what we end up with in terms of the delta h for the entire reaction here, is we end up with negative 2816, and it's going to be kilojoules per mole of glucose.
All right, so let's see how this matches up, and hopefully you can actually remember that this matches up actually perfectly here. So what we are going to see is that, in fact, what we calculated versus what is experimental is dead-on the same.
So there's actually one more way to figure out the enthalpies of a reaction, I'm going to go over it just very briefly. And that's based on the fact that enthalpy is a state function, and by state function what I mean is that it doesn't matter how you got from point a to point b, all that matters is the difference between the two. So another example of a state function is altitude, for example, on a mountain. So if you're talking about altitude, you go from point a to point b on the mountain, and it doesn't matter how you got there -- you could have climbed all the way up the top of the mountain, then went back down and eventually landed on point b. Or you could have gone straight from point a to point b. The change in altitude between point a and point b do not depend on the path, they're independent of path. So this difference right here does not matter on how you got there, it does not make a difference how you got there, altitude is a state function.
So similarly, we can say that enthalpy is a state function as well, if we're talking about part reaction a here, which has our reactants going to our products in 2 b here. It doesn't matter how we got there. We can actually calculate the change in enthalpy at all different points here. All that we actually have to worry about at the end of the day is the difference between a and b. So that's what we mean by state function.
Actually, we're not going to have a chance to get to fully explaining the consequence of this, which is Hess's law, which allows us to add and subtract different reactions. So what I'm going to say is the last two problems on your problem-set you won't have to do because we're not going to get a chance to cover in class on Friday. So I'll send out an email about this as well, so that'll be pushed back. So, it will be on the exam, so you want to do them at some point, but you don't need to actually turn those in. So those are the last two problems, I believe.
Free Downloads
Video
- iTunes U (MP4 - 111MB)
- Internet Archive (MP4 - 111MB)
Free Streaming
Subtitle
- English - US (SRT)