Flash and JavaScript are required for this feature.
Download the video from iTunes U or the Internet Archive.
Topics covered: Lewis structures
Instructor: Catherine Drennan, Elizabeth Vogel Taylor
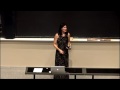
Lecture 11: Lewis Structures
Related Resources
Lecture Notes (PDF)
The following content is provided under a Creative Commons license. Your support will help MIT OpenCourseWare continue to offer high quality educational resources for free. To make a donation or view additional materials from hundreds of MIT courses, visit MIT OpenCourseWare at ocw.mit.edu.
PROFESSOR: All right, let's get started. Could everyone take 10 more seconds on the clicker question. And as a reminder, hopefully I don't need to remind any of you, but exam 1 is on Wednesday, so rather than our clicker question being on something from last class, which is exam 2 material, let's just make sure everyone remembers some small topic from exam 1 material, which is the idea of angular nodes. So I was hoping to see more like 99% on this, but you do still have two more days before the exam. Remember, we're talking about angular nodes here, so you need to read the question carefully. For an angular node, we're just talking about what the l value is, so whatever l is equal to is equal to the number of angular nodes you have. For an f orbital, what is the quantum number l equal to? Three. Good, so everyone that recognized that probably got the right answer of three angular nodes here.
So let's switch to today's notes. Two more quick things about the exam, the first is that just remember on Wednesday, don't come here, the exam is not here, don't come here. It's in Walker, so make sure you go to Walker. And also, keep in mind that I have office hours today from 3 to 5. All your TA's have either already have their extra office hours, or there are some that will be going on tonight or tomorrow, so keep those in mind as your finishing up your studying for the exam.
So, today we're moving on, we're talking about Lewis structures. This is a really good topic to do the class before an exam because it's a little bit of a lighter topic. We remember that Lewis structures are an idea that are pre-quantum mechanics. So that means that we don't have to worry about things like wave functions when we're talking about Lewis structures, but because they're so simple to use and because they so often predict the electron configuration of molecules accurately, we end up using them all the time in chemistry, so it's very valuable to know how to draw them correctly and to know how to work with them. So we'll talk specifically about drawing Lewis structures and then about formal charge and resonance, which are within Lewis structures.
So remember, that when we talked about Lewis structure, the organizing principle behind Lewis structures is the idea that within the molecule the atoms are going to arrange their valence electrons, such that each atom within the molecule has a complete octet or full outer shell. So this is the idea of the octet rule that Lewis came up with way back in 1902.
So, at the end of the class on Friday, we saw that list of eight steps that you always need to go through when you draw a Lewis structure. Once you're doing this on your own, especially, for example, on exam 2, which is a ways down the road, you won't be able to look at those steps, so you need to make sure that you can go through them without looking at them, but for now we can look at them as we are actually learning how to draw the Lewis structures, and rather just go through them step-by-step, it's more interesting to do it with an example.
So let's hydrogen cyanide as our first example. So we have h c n. So, in this example here, we start with the first step -- the first step in any Lewis structure is drawing the skeletal structure. So, essentially drawing how the atoms are arranged within our molecule. And in this case we have three choices here in terms of what's going to be in the middle, so we need to decide that first.
In terms of where different atoms are in a molecule, if you have a hydrogen atom or a fluorine atom, you can pretty much guarantee they're always going to be terminal atoms. By terminal I mean they're only bonded to one thing. So, for example, hydrogen or fluorine they'll never be in the middle, they'll always be on the end of a molecule.
So that takes care of the hydrogen, what about between the carbon and the nitrogen? In terms of picking a Lewis structure that's going to be the lowest energy, what you want to do is put the atom with the lowest ionization energy in the center of your atom. This should make sense because if something has a low ionization energy, that means it's not very electronegative, which means it's going to be a lot happier giving up electron density, which is essentially what you're doing when you're forming covalent bonds is you're sharing some of your electron density. So, we keep the atoms with the lowest ionization energy in the center.
So, why don't you go ahead and tell me, keeping that in mind, which atom in terms of h c or n would you expect to be in the center of hydrogen cyanide? And I put a periodic table up there, just the part you might need to look at. So this should be fast, so let's take 10 seconds.
All right, good job everyone. So most of you saw that carbon should be in the center. Carbon should be in the center because it has the lowest ionization energy. We know that ionization energy is going to increase as we go across the periodic table, so that means carbon has a lower ionization energy than nitrogen, which is right next to us. So as I just said, we want to put that one in the middle. You got an extra hint here in terms of the order, so even if you had just forgotten what I said, sometimes it's not a terrible idea just to put it in the order it's written, that can give you a lot of clues as well. So, either of those ways of figuring this out is the first guess of what goes in the middle will work pretty well.
So, let's go ahead and draw our Lewis structure based on the rest of the rules now that we have a skeleton. So our skeleton tells us that carbon is in the middle, so we'll put the h on one side, and the n on the other side there. So, our second step, as we go through our Lewis structure rules, is to figure out how many valence electrons we have in our entire molecule. So if we talk about hydrogen, how many valence electrons are we talking about? 1. What about carbon? I heard 4 and 6. And remember, we're only talking about valence electrons, so the outer-most shells. So we're talking about four valence electrons for carbon. And then for nitrogen? Lots of options I have to choose from from these answers, but it's 5. So, if you can't immediately know, and you don't all have periodic tables in front of you, so that's fine, but if you have a periodic table in front of you, you need to be able to count valence electrons, so work on that if it doesn't come naturally to you in terms of figuring that out.
So then in order to figure out the complete number of valence electrons in our molecule, we just add 5 plus 4 plus 1. So we end up having 10 valence electrons.
Step three in our Lewis structure rules is to figure out how many electronis we would need in order for every single atom in our molecule to have a full valence shell. So, if we're talking about hydrogen, that's our one exception so far to the octet rule. So we actually only need two electrons to fill up the valence shell of hydrogen, remember that's because all we need to fill up is the 1 s.
However, for carbon and nitrogen we need 8 each. So in terms of total numbers that we would need to complete our octets and fill our valence shells, we would need 18 electrons.
All right. So let's bring down our middle slide here. So we have 18 electrons, and the next thing that we need to figure out is how many bonding electrons we have. So to figure out bonding electrons, what we take is that number 18, which is our total number of electrons we need to fill valence shells, and we subtract it from our number of valence electrons, which is 10. And what we find that we have is 8 bonding electrons.
And hopefully on your paper, you can actually reach your h c n skeleton -- I think I should probably re-draw mine here. Because step five is that we need to fill in our bonding electrons, and we start it with filling in two electrons per bond. So I'm just going to re-draw my skeleton. So, the first thing we do is put two electrons between h and c, and then two electrons between c and n. Remember, every time we have two electrons that are being shared, that's a single bond.
The next thing that we want to do is figure out do we have any bonding electrons left. So let's see, we started with 8 bonding electrons, and we used up only 4, so the answer is yes, we have 4 bonding electrons left. So what we need to do is fill in those extra bonding electrons into our bonds. Should I put an extra pair of electrons here, does anyone think? No. The reason is because we already have a full valence shell for our hydrogen, it doesn't want anymore electrons. What about between the carbon and nitrogen? Yes. Definitely, because both of these are not anywhere near filling up their octets yet. So we can put actually all 4 of our extra electrons in between the carbon and the nitrogen. Now we have 6 things around the nitrogen, and we have 8 around the carbon.
So, what we do as our seventh step is then figure out if we have any extra valence electrons left at all. So we started with 10 valence electrons, we used up 8 of those electrons in terms of making bonds. So it turns out that we have 2 valence electrons left. So we need to add those 2 valence electrons left as lone pair electrons in our structure.
So, which atom is in need of those lone pair electrons? The nitrogen. The reason being that's the only one that didn't have a full octet yet.
So now we're done, actually there is one more step, which is to determine the formal charge. This is a good way to actually check if your Lewis structure is correct or not. We haven't actually learned how to calculate the formal charge yet, we'll learn it soon. So we won't do it for this molecule, but we'll go back and do it for some of our other examples, and you can go back and do it for this one.
The other thing is that we can re-write our h c n in terms of bonds. So we know every time we have two electrons, that's a bond. So we have h, then we can draw our bond as a line. And then we have a triple bond there because we have 3 pairs of electrons. So it looks a lot less messy if we just draw our Lewis structure like this for h c n, where we have h bonded to c triple, bonded to n, and then a lone pair on the nitrogen there.
All right, so this is the same procedure that we're going to go through, regardless of what kind of Lewis structure we're going to draw. What you'll actually find in terms of asking your TAs about the Lewis structure rules is that sometimes they won't be as good at them as you are, and the reason is once you've drawn enough of these structures, you start to get a lot of chemical intuition about what's right or what's not right -- it just looks wrong to you if it's wrong. So your TA might take a minute, so be patient with them if they see your structure and they say oh, no, no, no, no that's wrong, that's terrible, and they don't immediately know why. They might need to go through the rules with you, you might need to remind them. Hopefully, they'll all study them again, so this will be an issue.
But what really happens is as you go on in chemistry, you draw so many of these you can just draw them without following the rules. Some of you might get almost to that point, or you might be at that point now, but I recommend this for you and for me and for the TAs, go through the rules because there'll be cases where it's a little bit tricky and it's always much faster to have gone through step-by-step, than to try to just kind of hit or miss figure out what's going to be right or wrong.
So, let's try another example here, and let's try a case now where instead of dealing with a neutral molecule we have an ion, so we have c n minus. And what I'll mention to you just in terms of the fact that we're finally dealing with real molecules, which is -- or molecules that are made up of more than one atom, which is kind of exciting for me and maybe for some other of you that like to move into thinking about what some of the consequences of these molecules reacting might be. A lot of the examples that we're going to give you in terms of trying out your Lewis structures will be molecule that are used in organic synthesis, or maybe they're molecules that react in interesting ways with biomolecules in your body or proteins in your body. So, you already will have a head start when you get on to later classes, like organic chemistry or if you're thinking about biochemistry where being able to draw the Lewis structure allows you to think about, eventually, the reactivity of the molecule, which becomes very interesting in thinking about how you're going to synthesize a more complex molecule, or how that molecule is going to interact with an active site in a protein in the body.
So, for example, just talking about hydrogen cyanide or the cyanide anion, these are both molecules which are used in organic synthesis, so particularly the cyanide, anion and salts of the cyanide anion. So either a potassium cyanide or sodium cyanide, these are used in synthesis in terms of making carbon-carbon bonds. So if you're trying to make a more complicated organic molecule, carbon-carbon bonds are one of the most difficult things to make an organic chemistry, and it turns out that c n minus is a very reactive molecule, so it's a good way, even though we'll go over some drawbacks in a second, it is a good way to make carbon-carbon bonds. It's very reactive. And because, of course, we have this carbon here what you end up doing is adding a carbon to your molecule.
So, when you think about cyanide, you might not think about organic reagents. Does anyone have something else they think about when they think about cyanide?
STUDENT: Death.
PROFESSOR: Death -- that's a good thought. Yes, cyanide and death, very closely related as well. Cyanide is incredibly toxic, it's a poison. That might be how you're more familiar with cyanide. So if you're working with cyanide in the lab as potassium cyanide or sodium cyanide, those are what are called p h s's, or particularly hazardous substances -- it's a rating for different kinds of chemicals. And what that means it's there's all sorts of precautions and procedures you take that are special when you deal with these. They're kept away from other chemicals. You handle them very special in terms of being extra careful in a very high ventilation area, in hoods is how you handle them. So, yes, they're very poisonous, and in fact, there are areas where you find this toxic compound, cyanide. Other than just in poisons and in organic synthesis shells, you might also find them in some things we're more familiar with, such as almonds. I don't know how many know that there are trace , trace amounts, of cyanide in almonds. I don't know if there any big almond eaters out there. You don't have to worry, we're definitely talking about trace, trace amounts. It's not going to hurt you. And actually, what we usually eat are what are called sweet almonds, and there aren't actual cyanide in the sweet almonds we eat, there's precursors to cyanide, which might not make you more comfortable. But the fact is there are trace, trace amounts. This is nothing that we need to worry in our food supply.
However, some people aren't so lucky. I don't know how many of you are familiar with the Cassava plant, which is a kind of woody shrub that's first been cultivated in South America, but it's grown throughout Africa, the Caribbean, South America still, many places around the world, and this is a major source of carbohydrates for much of the world, because Cassava root is very, very rich in carbohydrates. It's not the best form of food in that they're actually very poor in protein, and unfortunately very, very rich in cyanide. So, these roots can be very dangerous. There's different types of the root that you can get called the bitter and the sweet. Hopefully you would all choose the sweet if two are put in front of you.
The bitter, of course, are the ones that are very high in cyanide. If you eat these raw, which they do in many places around the world, if you eat a bitter one, you could, in fact, get enough cyanide to kill you. And there are ways to prepare these, so it's important -- this kind of thinking more along the food science idea. There's a way to actually grind down and prepare the flower, so that you promote the enzymes within the plant to breakdown the cyanide precursors. And if you put this in the well-ventilated area, if you prepare this outside, the h c n gas will actually be released into the air, so you're safe, you can eat it later. About 80% of the cyanide at that point is gone, so it does render the root much more safe.
But you do, in fact, have to worry about long-term exposure, cyanide poisoning in terms of long-term effects in certain populations that do get the bulk of their carbohydrates from this root, from the root of the Cassava plant.
But in terms of us going to the grocery store and thinking about things, probably we're all breathing sighs of relief. I just told you almonds are not a problem, no worries there. We probably don't find any forms of the Cassava plant ever in the U.S. that are going to have that high cyanide content, so we should all be relieved. Unless, of course, you're a smoker, or you're thinking of becoming a smoker, and then maybe you should worry, because this is one of the advertisements that was airing in terms of anti-smoking campaign. Hydrogen cyanide is found in cigarettes. So, if you're looking for another reason to quit, if you're looking for a reason not to start smoking, here's another good one. As I said, it's a particularly hazardous substance, this is worked with in fume hoods. You don't want to inhale it, it's definitely not recommended. The way, in the simplest terms that cyanide can kill you, is it basically out-competes your oxygen for the heme in your blood. So instead of carrying oxygen to your cells, you're carrying cyanide to your cells. Obviously, the amounts that are in cigarettes are not enough that people are dropping dead of cyanide poisoning, but still it's not a good idea if you can avoid eating or inhaling cyanide -- you definitely want to minimize your exposure.
And in terms of thinking about it for organic chemistry or if you're interested in thinking about the mechanism maybe by which it is toxic, a first step would be to draw its Lewis structure. So, let's go ahead and make sure we can draw that, if we have interest either in the area of organic chemistry or biochemistry or biology here. So in terms of the first step of skeletal structure, this is actually going to be easier because we don't have a central atom, we just have carbon and nitrogen here.
Our next step is thinking about valence electrons. So we have 4 plus 5, but we're actually not done yet, because it's c n minus, so if we have minus, we actually have an extra electron in our molecule. So we need to add 1 more. If instead we had a positive ion, a cation, what we would have to do is subtract 1. But here we're going to add 1, so again, we have 10 valence electrons. And if we go on to step three where we figure out how many we would need for full octets, it's just going to be 2 times 8, so we have 16. And step four is going to have us figure out how many bonding electrons we have, so we have 16 minus 10, is going to be 6 bonding electrons.
So, step five tells us to add 2 electrons between each atom, so we add two there. And step six asks us, well, do we have any bonding electrons left? So how many bonding electrons do we have left? Yup, so we do, we have 4 left. We started with 6, we only used 2. This is very easy molecule because we know exactly where to put them without even having to think, we only have one option, and we'll make a triple bond between the carbon and the nitrogen.
So, seven asks us if we have any valence electrons left, and how many valence electrons do we have left? Yeah, so also 4. We started with 10 valence electrons, we used up 6 of those as bonding electrons, so we have 4 left, which will be lone pair electrons. So, in order to fill our octet, what we do is put two on the nitrogen and two on the carbon.
So, in terms of finishing our Lewis structure, we're actually not done yet here, even though we have full octets, and we've used up all of our valence electrons, and the reason is because it's c n minus, so we need to make sure that that's reflected in our Lewis structure, so let's put it in brackets here, and put a minus 1.
And also I wanted to mention in terms of checking your Lewis structures, regardless of what they are, you should always go back and say how many valence electrons did I have -- I had 10, and then count 2, 4, 6, 8, 10, because you always need to make sure you have the same number of valence electrons that you calculated in your actual structure. That'll catch a lot of just silly mistakes for you if you go back and see it and you don't have all of that.
Let's re-draw this, so it looks a little bit neater, where we have a triple bond in the middle instead, and again, we need our negative 1 charge there.
And our eigth step in the process, again, is formal charge, which we will talk about very soon.
All right. So let's try one more example of drawing Lewis structures before we talk about formal charge. And the last example that we're going to talk about is thionyl chloride, so it's s o c l 2. This is another good step forward, because now we actually have four different atoms in our molecule. I'll tell you a little about thionyl chloride as well. This is another organic chemistry reagent, it's also used extensively in the pharmaceutical industry. And what it's used is to convert one type of group, what's called a carboxylic acid into another type of very reactive intermediate, which is called an acid chloride. So I show that here, so in green, you have what's called a carboxcylic acid group, a c o o h, which gets converted by s o c l 2 to a c double bond o c l or an acid chloride. This is the very reactive intermediate. You'll learn a lot more about this if you take organic chemistry, but, In fact, you can then go on and make a bunch of other different kinds of very interesting molecules.
So, for example, this is the synthesis of novacaine. This is what's used in industry to actually make novacaine. Has anyone had a novacaine procedure? Yes. I've had it also many times, you usually get novacaine for cavities. There's some alternatives that are used now as well. It's also used as a local anesthetic for other types of small procedures.
So, this is, in fact, what's used to make novacaine in industry. You'll notice that a lot of different kinds medications do you have chlorine in them, you'll see that c l group. So, for example, you might be familiar with Wellbutrin here, this is a type of anti-depressant that a lot of people use right now that are taking anti-depressants. It's on the market, very popular in terms of your choices right now as an option as an anti-depressant. Also, Lunesta. This was very big in an ad campaign at least last year, I'm not sure if it still is, with the little butterfly. This is the structure of Lunesta, and you see the c l in it as well. I just wanted to point out that although you see these chlorine atoms in these drugs, what you almost never see is an acid chloride -- in fact, I don't think I've ever seen an acid chloride in a final pharmaceutical product or drug that we take, and the reason is because they're so reactive that you wouldn't want to have that in something you digest. So just keep in mind when you do see the chlorine in these drugs, it's very different from the acid chloride. So, for example, Wellbutrin, it is very unlikely that it would have thionyl chloride in order to make it, and if thionyl chloride was used at some point in the synthesis, it was not to put that chlorine atom on, it was to put something else on.
But in terms of drugs that don't look like maybe this compound was used in the synthesis, many of them might have used thionyl chloride, because it generates such a nice reactive intermediate that you can go on and make a bunch of different compounds from that intermediate.
All right. So let's think about how to draw the Lewis structure for thionyl chloride -- oh, actually, let me let you tell me how we should start this Lewis structure. So, which atom would you expect to be in the center of a Lewis structure for thionyl chloride? All right. Let's take 10 seconds on that.
Looks like we have some fast thinking here, a lot of last minute answers coming in. OK. We have a split decision, so -- you know what, actually, let's think about this for a second. So hopefully, it was a time issue in terms of looking at the periodic table, because let's have you tell me what are we looking for here? Yeah. OK. We're looking for the lowest ionization energy. So, this one can be tricky because oxygen looks like it's in the middle because of the way it's written, but we need to start by looking at the lowest ionization energy.
So, if we look on the periodic table, comparing, for example, s to o, if we have s it's below o, what happens to ionization energy as we go down a table? It decreases. If you're still not completely up on the periodic trends, that is stuff that's going to be on the first exam, so make sure that you're able to do this without taking too much time to think about it. We would expect the ionization energy to decrease, that means that sulfur has our lowest ionization energy.
All right. So, let's go ahead and draw our Lewis structure here with sulfur in the middle. So, we can put our sulfur in the middle, and then it doesn't really matter how we draw the rest of it, where we put our c l's versus where we put our oxygens. We'll just put them in some way around our sulfur atom. So that's our step one.
For our step two, what we need is number of valence electrons. So we have 2 for each of the chlorine. How many valence electrons are in chlorine? All right. So it's 7 that are in chlorine, it's the same as fluorine or any of the others in that row or in that group rather. 2 times 7, plus we have 6 in the sulfur, and oxygen is right above sulfur, so that also has 6. So we end up having 26 valence electrons that we're dealing with here.
Our step three is to figure out how many bonding electrons that we need, or excuse me, how many total electrons that we need to fill up our octets, so that's just going to be 4 times 8, which is 32. And then we take 32 minus 26. So what we end up with in terms of our bonding electrons is going to be 6 bonding electrons. So we can go right ahead and fill these in. 1 2, 3 4, 5 and 6. And that was step five.
Step six is thinking about do we have any bonding electrons left? Nope, we used them all up. So we don't need to put any more bonds in there.
And step seven is how many electrons do we have left over that are going to go into lone pairs? How many? 20. 26 minus 6, so that tells us 20, and these are all going to be lone pairs. Well, we're talking about a pretty high number here, so to make counting easier, we'll just say 10 lone pairs, because 20 lone pair electrons is the same thing as 10 lone pairs. And all we need to do is go over here now and fill up our octets. So oxygen gets 3 pairs, and each chlorine gets 3 pairs, so now we're up to 9 pairs. And what we have left here is the sulfur, which will also get a pair. So, if you look at all of these, we have full octets for all of them, and if we count up all of the valence electrons, it's going to be equal to our number 26 here.
And the last thing we do for any of our structures to check them and figure out are these valid or not valid, are these good Lewis structures is to check the formal charge. So now that we have enough practice drawing Lewis structures, let's talk about actually figuring out this formal charge.
So when we talk about formal charge, basically formal charge is the measure of the extent to which an individual atom within your molecule has either gained or lost an electron. So as we said when we first introduced covalent bonds, it's a sharing of electrons, but it's not always an equal sharing. Sometimes we have a very electronegative atom that's going to take more of its equal share of electron density. So for example, that might have a formal charge of negative 1, because to some extent it has gained that much electron density that it now has a formal charge that's negative.
So, when we think about any type of formal charges, we have to assign these based on a formula here, which is very easy to follow. Formal charge equals v minus l minus 1/2 s. It's even easier to follow if we know what all of those stand for. So, f c, I think you all know is formal charge. Does anyone have a guess for v? Everyone has a guess, great. Valence electrons. What about l? Lone pairs. So, lone pair electrons, actually, not lone pairs themselves. And then s? Good. That's a tricky one, shared electrons. All right. So this means we can actually calculate this for any molecule that we've drawn the Lewis structure for, because we actually do need to draw the Lewis structure before we know, for example, how many of each of these we have, or at least go through the rules.
And what's important to keep in mind about formal charge is if we have a neutral atom, such as we did in thionyl chloride here, the sum of the individual formal charges on individual atoms within the molecule have to equal 0. So if we add them all up, there should be no net charge on the molecule, if the molecule is neutral.
So, if we think about the second case here where we have c n minus, now we're talking about a molecule with a net charge of negative 1. So that means if we add up all of the formal charges within the molecule, what we would expect to see is that they sum up to give a net charge of negative 1. So we can do this for any final charge we have, if we a molecule that has a charge of plus 2, then all of the formal charges should add up to plus 2 and so on.
So, let's just figure this out for some of the examples we did, so for the cyanide anion. So, if we want to figure out the formal charge on the carbon, we need to take the number of valence electrons, so that's 4. We need to subtract the lone pair, what number is that? It's 2. And then 1/2 of the number of shared electrons. So, shared electrons are the ones that are shared between the carbon and the nitrogen, so we have 6 shared electrons, and we want to take 1/2 of that. So we end up with a formal charge on carbon of negative 1.
We can do the same thing for nitrogen. So in terms of nitrogen that starts off with a valence number of 5, again we have 2 lone pair electrons in the nitrogen, and again, we have 6 electrons that are shared. So what we see is that the formal charge on the nitrogen is 0. Also, formal charges can be checked, as I just said. Negative 1 plus 0 should add up to negative 1, if in fact, we're correct for the c n anion. And it does, so we know that we're probably on target in terms of calculating our formal charge.
So, let's think about our second example -- actually our third, but the second one we're going to talk about in terms of formal charge, which is thionyl chloride. So why don't you tell me what the formal charge should be on the sulfur atom of thionyl chloride?
All right. Let's take 10 more seconds. OK, so the majority got it. So hopefully next time we do a formal charge question, we'll get everyone back up to speed. But we've just introduced it, so let's go back to the class notes and explain why this is the correct answer. So if we look at sulfur, what we need to do is take the valence electrons in sulfur, and there are 6. By looking at the periodic table it's right underneath oxygen, so those both have 6 valence electrons. There are 2 lone pair electrons on sulfur -- we only have 2 lone pairs -- or 1 lone pair, 2 lone pair electrons. And then we end up having 6 shared electrons, 2 from each of the bonds, so we end up with a formal charge on sulfur of plus 1.
If we go to the oxygen atom, now we're talking about starting with 6 in terms of valence electrons again, but instead of 2, you can see we have 6 lone pair electrons around the oxygen minus 1/2 of 2, so we have minus 1 is our formal charge. And if we talk about chlorine, and both of the chlorines are the same in this case, we start with a valence number of 7 for chlorine, and then we subtract 6, because it had 6 lone pair electrons around each of the chlorine atoms. Then minus 1/2 of 2, because we only have one bond or 2 electrons in a bond.
So again, we should be able to check all of our formal charges and make sure they add up to 0, which they do, and that makes sense, because we have a neutral atom in terms of thionyl chloride.
Another thing to mention in terms of thinking about if you have a good or a bad Lewis structure, is that when you figure out the formal charge on each of the atoms, it's the more electronegative atoms that you would expect to have that negative charge, and that should make sense to you because electronegative atoms want to have electron density, they want to pull in electron density to them, so it would make sense that they have more of it, which would give them a negative charge.
So, if we compare the sulfur to the oxygen, the oxygen it turns out is more electronegative and that is what holds the negative charge in this molecule.
Another thing I want to point out, and for some of you just ignore what I'm saying, if you haven't thought about oxidation number, if you haven't heard of that before, don't worry we'll get to it in the second half with Professor Drennan. But for those of you from high school that have learned about oxidation number and maybe are starting to think about it when you look at these molecules, formal charge is -- it's not the same thing as oxidation number, so separate those two things in your head. We'll get to oxidation number in the second half of this course, but it's not in any way the same idea as formal charge.
All right. So formal charge can actually help us out when we're trying to decide between several Lewis structures that look like they might be comparable in terms of which might be the lower energy or the more stable structure. The examples we've done so far have been pretty straightforward, so we haven't needed to use formal charge to make this kind of decision. But we could, for example, look at a case where we have several different structures that look pretty good, and the one we want to determine as being the lowest energy structure is the one in which the absolute values of the formal charges are going to be lower, so essentially that they have less charge separation. Those are going to be the more stable or the lower energy structures.
So, for example, let's look at thiocyanate ion, we have c s and n. What we've learned so far is as a first approximation, what we want to do is put the atom with the lowest ionization energy in the middle here. So let's compare those ionization energies. We have 10 90 for carbon, 1,000 for sulfur, and 1,400 for nitrogen. So, thinking about ionization energy, which atom would you put in the middle here? So, a lot of people I hear are saying sulfur, and that's right. So, in terms of ionization energy, we would expect to see sulfur in the middle. So if we went through and drew out our Lewis structure following each of our steps, what we would get is this as our Lewis structure here, and we could figure out all of the formal charges. But what I'm going to tell you already is this is a case where, in fact, it's an exception to the idea that the lowest energy structure has the lowest ionization energy in the middle, and we can figure this out when we look at formal charge. It's always a good first approximation, because you need to start somewhere in terms of drawing Lewis structures, but then if you go and figure out the formal charge and you just have lots of charge separation or very high charges, like a plus 2 and a minus 2 and a minus 1 all different places in the atom, what it should tell you is maybe there's a better structure.
So, let's think of all of the combinations that we could have in terms of this molecule. So in one case, we could actually put carbon in the middle, in one place, we could put sulfur in the middle, and in one case we could put nitrogen. And then we can go ahead and let's quickly write out what the formal charges for all of these will be. So in our first structure, we would find for the nitrogen we have a formal charge 5 minus 4 minus 2, because we're starting with 5 valence electrons, so that is a formal charge of minus 1.
For the carbon, we start with 4 valence electrons, we have 0 lone pair electrons minus 4, and we end up with a formal charge of 0. For the sulfur, we start off with 6 valence electrons, minus 4 lone pair electrons, minus 2, taking in account our bonding electrons, so we end up with a formal charge of 0.
All right, so this looks pretty good. We don't actually have much charge separation in this case here. Let's take a look at the lowest ionization energy in the center case. And what you find out if you do these calculations, is that you have a negative 1 for your formal charge on nitrogen, you have a negative 2 for your formal charge on carbon, and you have a positive 2 for your formal charge on sulfur.
If we look at our last structure here where we have nitrogen the middle, we can also figure out all those formal charges, and in this case we have plus 1 on the nitrogen, we have minus 2 on the carbon, and then we end up with a 0 on the sulfur there.
So let's go to a clicker question. If we call this structure a, b, and c -- you can look on your notes, it also says structure a, b, and c on your notes, so you didn't need to have just memorized that slide. But I want you to tell me in terms of thinking about formal charge, which Lewis structure would you predict to be the most stable? And this should be fast, so let's take 10 seconds on this.
Okay, great. So let's go back to our notes here. And the reason that this should be so fast is we already did all the calculations for the formal charges. So what we see is that structure a is the most stable because we have the least separation of charge in the case of structure a. And you can do this any time if you have Lewis structures that you're choosing between. You won't always draw out every single possibility that you have to start with. Often a good thing to start with is to put the lowest ionization energy atom in the middle, and if you don't have charge separation then go with that structure, but if you do find you have a lot of separation, such as the case in negative 2, positive 2, and minus 1, then you want to say wait a second, this is really bad in terms of formal charge, let me go ahead and see what other options I have here.
So, we can also get into a case where we have similar values in terms of absolute values of formal charge between two different molecules we're deciding between in their Lewis structures. And in this case, the tie-breaker goes to the molecule in which the negative charge is on the most electronegative atom.
So, let's look at an example of this here. So we could say, for example, this molecule here, this -- now we're dealing with a lot of different atoms in the molecule, much more complicated than the initial case of the cyanide ion where we only had two. So, how do we figure out first how to draw the skeletal structure of this molecule here? And one thing I want to tell you to start out with is something about this c h 3 group here. Any time you see a c h 3, this means a methyl group. And if you draw out what a methyl group is -- hopefully you won't have to spell it -- then what you have is a carbon in the middle with three hydrogens around it, and then it can only be bonded to one other thing. So any time you see c h 3 here, remember that that's methyl and that's going to be a terminal group. So, you already have a hint that methyl groups are never in the middle, they always have to be on the outside. So that's a good start for us putting together a skeletal structure for this compound here. The other tip I'm going to give you is any time you see a chain molecule, by chain I just mean many different atoms written out in a row. A convention is that typically you will put a terminal atom. We know that h is always terminal, it's always on the end, never in the center. Right after the molecule that it's attached to. So, for instance, this would suggest to us by the way it's written, that the hydrogen is attached to the nitrogen and not the oxygen.
So, if we use those two tips to try to figure out a structure, a skeletal structure, we would get this structure here if we write out the full Lewis structure. We could I think well, maybe this isn't written out in terms of that convention, which sometimes it's not, so let's also try writing it, such that we have the hydrogen and the oxygen atom there. So that would give us this structure here. So notice a difference in these structures, is this has an n h bond whereas this has an o h bond. And if we were to think about which one of these is better, it turns out that it's the same in terms of formal charges, so that doesn't help us out. So we need to go to this second case where we're instead going to think about electronegativity, and we want to think about which atom is the most electronegative.
So, in this case, we see that our formal charge is negative on the nitrogen, in this case it's negative on oxygen. Which of those two is more electronegative? The oxygen. So you should be able to look at your periodic table and see this, or also I've written the trend here. So that means that the more stable molecule is going to be this molecule here, which actually puts the negative charge on be more electronegative atom. So this is our lower energy structure.
So, these are the different ways that we can actually go ahead and use formal charge when we're choosing between different types of Lewis structures.
So one last concept that I want introduces is this idea of resonance. And resonance is the idea that sometimes one single Lewis structure does not adequately describe the electron configuration around a given molecule, so instead you need to draw two different Lewis structures to describe that more appropriately. So, let's quickly go through the Lewis structure for ozone. I have the skeletal structure written up there, I've written it twice and you'll see why in a minute. It's easy to write the skeletal structure, because it's all oxygen, we don't have to worry about what's going to go in the middle.
In this case, we're going to have 3 times 6 for valence electrons -- 6 valence electrons for each oxygen, so we have 18 total valence electrons. So, in order to fill up our shell, what we need is 3 times 8 or 24 electrons. This leaves us with 24 minus 18, or 6 bonding electrons left. So what we can do is fill that in here. Clearly, we put 2 for each bond, and now we end up having 2 remaining bonding electrons left. So here is where our question comes, because where do we put it? There's absolutely nothing that tells us which atoms we should put it between, because they're both oxygen-oxygen.
So, let's just arbitrarily put it between these two in this case here, but actually there's no reason we couldn't also put it between oxygen b and c, so I'm going to draw another structure where we have it here. So in terms of remaining valence electrons we have 12, so we can finish off each of our Lewis structures, so that's our first structure there, and our second structure there. We could also figure out the formal charges, and obviously the formal charges between these two atoms, they're going to be identical, we're only dealing with oxygen atoms here.
So, we need to think about what this means -- which is the more stable structure, because we have two different structures here. In this case we have a double bond between a and b, and in this case we have it between b and c. So, presumably, if we follow our rules so far only one of these should be correct. And again, if we figure out the formal charges, let's go through this quickly, we get 0 plus 1 and negative 1 for structure 1. We get negative 1 plus 1 and 0 for structure 2. So as I said, they're going to be identical in terms of making the decision that way.
And what it turns out is experimental evidence tells us that these two structures are equivalent. And by that what we mean is that they're absolutely identical, and it turns out that this here is not a double bond, it's not a single bond either, it's actually something in between. So if we look at its length, it's actually shorter than a single bond, but longer than a double bond. Or if we look at how strong it is, it's actually stronger than a single bond, but weaker than a double bond. And we find the same thing for these two atoms here, it's not actually a double bond, it's somewhere between a single bond and a double bond.
So this is a case where we have resonance structures, or we call this a resonance hybrid. So the reality of the situation is that it's a combination between these 2 structures. And an important thing to remember when we talk about resonance hybrids is that the structure it's not 1/2 the time this structure, and 1/2 of the time this structure, it's actually some combination or some average between the two structures. But since in drawing Lewis structures we don't have a way to represent that -- actually, in some cases you do, you can draw a dotted line that means a 1 and 1/2 bond, but most in most cases, we just draw out both resonance structures, and the way that we say it's a resonance structure is that we put it in the brackets and we put an arrow between it.
So, when you think about resonance structures, some students tend to just get confused and be picturing this flickering back and forth. A good example to keep in mind is the idea of a mule. So most of you know, hopefully, that a mule is a combination of a donkey and a horse. A mule is not spending 1/2 of its time as a donkey, and 1/2 of its time as a horse, we don't see it flickering back and forth between the two, that's not what we see. Instead what we see is it's an average, it's part like a horse, it's part like a donkey. So if we want to put that in chemical terms, we want to make sure we put these in brackets here, and remember, this is the resonance arrow, it's not a reaction arrow, it's a resonance arrow, so make sure you mark it up correctly like that.
When we talk about resonance structures, the key word is that the electrons are de-localized. So they're not just between two atoms here. Now, for example, in our structure with ozone it's between all three atoms.
And the final point I want to make today, and this is very, very important, so make sure that you do understand this. When we talk about resonance structures, we're talking about cases that have the same arrangement of atoms, the key is the atoms are the same, and the thing that is different is the arrangement of electrons here.
Free Downloads
Video
- iTunes U (MP4 - 112MB)
- Internet Archive (MP4 - 112MB)
Free Streaming
Subtitle
- English - US (SRT)