Flash and JavaScript are required for this feature.
Download the track from iTunes U or the Internet Archive.
Description: This lecture is the second of five on the hypothalamus and limbic system and focuses on their functions.
Instructor: Gerard E. Schneider
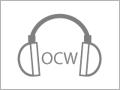
Lec 28: Hypothalamus and li...
The following content is provided under a Creative Commons license. Your support will help MIT OpenCourseWare continue to offer high quality educational resources for free. To make a donation or view additional materials from hundreds of MIT courses, visit MIT OpenCourseWare at ocw.mit.edu.
PROFESSOR: We had finished talking about the pituitary organ and what's being secreted there. Looked at it for a rodent and for a human. And I just got up to this question, number six there. What homeostatic mechanisms are associated with the hypothalamus? So what do we mean by homeostatic mechanisms? Anybody?
Usually we're talking about internal environment and maintaining something in a homeostatic fashion. It means "same state." But, of course, do we really mean "same state"? It would be almost impossible to maintain it, like glucose levels, for example. So instead, our bodies maintain levels of various things, including temperature, glucose levels, various minerals in our blood, and so forth, in a certain range.
And if it gets outside the range, then what happens? If it's temperature, we can think of things that happen automatically to raise temperature or to lower temperature, if our core temperature gets too far from that set range. And as we know, in endothermic animals like ourselves, that range is fairly small.
OK. And why do you think that is? Well, various biochemical processes work best in a certain range. And the brain functions best in a certain range. That's why the poikilothermic animals-- the exothermic animals that don't maintain their body temperatures in such a narrow range-- the reptiles, the amphibians-- they don't function well when it gets cool.
And it's not that they totally-- reptiles, in particular, will basically seek a quiet place where they can rest if it's too cool, and they do their hunting after they've warmed up. Like the iguana, they will go out on rocks, warm up quite a bit, go up into the upper range of temperatures that their body can tolerate. And then they go into the water. And the bigger ones can stay in longer than the smaller ones, because they don't lose body temperature quite as fast.
But then what do we do if-- what happens to our bodies if we get too cold? Well, it-- sorry?
AUDIENCE: Piloerection.
PROFESSOR: Piloerection for most mammals. It doesn't help much with us, but we still have that reflex. What else? Shivering, because that generates heat from our muscles.
But we do a lot more. Our behavior changes. We go inside. We put on clothes. We put on a coat, and so on and so forth. Well, animals do it also. They may not have clothes, but they've got usually better protection. But they seek warmer places.
I think rodents have a nice solution, the ones that live underground, because you go very far underground and the temperature is the same all the time. Now, we can say we maintain our blood glucose homeostatically, but we don't. I mean, a few people do, but most of us don't eat all the time. Some people are always snacking.
But normally we're just eating at certain mealtimes, because our body has various mechanisms for maintaining blood glucose. And, of course, we need various nutrients that we don't have to constantly take in. Some of them we need only every few days.
But, anyway, that, of course, involves a lot of behavior. So we mentioned temperature regulation. I think we've already defined all of this. But there's other cyclic behaviors besides eating, OK-- feeding and drinking. Animals that are predators have cycles of predation and rest and eating.
And all animals have a cycle of sleeping and waking. There's a lot of variation in it, and of course there's day-active and night-active animals. But they all have it. We know how it's-- we talked about how it's regulated through the visual entrainment of the biological clock.
And then we have episodic behaviors. That simply means there's not a regular cycle. But they're still under the control of the hypothalamus. They're still part of our biological drive equipment that centers on the hypothalamus, but involves a lot of other structures that are connected with the hypothalamus.
The agonistic behaviors. That means fighting and fleeing, or defending. Reproductive behaviors. And that includes sexual behaviors and parental behaviors. And we know that associated with these drives and the actions that result are various emotions.
So if the hypothalamus is stimulated in some way, you don't just affect these drives. You affect what we call "feeling"-- technically, they're called "affects"-- and the emotional displays, the actual actions. How do we show emotion? Mainly through facial expression.
What's its purpose? We can-- it's communicative. It's a social behavior. Other members of our species-- our mate, our friends-- know what we're going through.
All right. And in this diagram of the locomotor system, which we saw earlier, this is how the hypothalamus was simply depicted as part of a hierarchy of control of the action pattern we just-- in other words, it was a motor system. And at the top level, we have the drives.
And locomotion is connected with most of these motivational states that we are talking about. And because of that, the activity of hypothalamic systems, we know that these things are generated, not just from the external environment, but from changed level of motivation controlled by our brain. It's called endogenous control.
But the hypothalamus doesn't act by itself. There's many inputs to it. I'm just showing some descending inputs here, from the olfactory system through the striatum-- other systems, through the thalamus-- the older thalamus-- and there we see [? the newer ?] thalamus.
So that's the general picture that you-- it's useful to know this kind of thing when you're thinking of motor control. But now it plays a major role in thinking about how the hypothalamus works, because it's so critical to this.
We've talked, I think, already about appetitive and consummatory behavior involved in all of these motivational states. I discussed that a lot in the animal behavior class. But we've brought it up here. You should be able to distinguish them.
What you may not know, and I do discuss it in the chapter a little bit, is the way if you disconnect hypothalamus from the brain stem, but not completely, so these drives are still affecting behavior, but you can mess up the way these consummatory behaviors-- these actions-- are expressed. Many of them are complex.
So for example, in the cat feeding behavior, the cat doesn't just eat-- a cat in the wild-- I mean, he has to catch prey. He has to take the prey to where it's safe to eat it. Some of them take the prey up a tree. They usually drag them away from the site where they killed it.
If you've got a pet and the pet is just fed, what happens to all that behavior? Well, he normally suppresses it. But if you disconnect, at least partially, you partially disconnect the hypothalamus from those downstream mechanisms-- separate the hypothalamus from the midbrain, but only partially-- you can get really bizarre patterns.
You can get cats-- they're pet cats, or at least tame cats. They may be laboratory cats, but they're taken care of pretty much like pets. They interact with humans a lot. They can totally change their behavior.
They can start treating every bit of food like they had to kill it. And they'll pounce on it, execute a killing bite. If they see water, they'll show fishing behavior. Well, we don't even see that much in pet cats, unless you have goldfish at home, and occasionally that innate behavior of cats will appear.
But after these types of lesions, you get bizarre combinations. And you also get some that are just missing. You know, things they just leave out, because the hypothalamus affects the probability of all these different behaviors connected with the drive, OK.
And if you're disconnecting different aspects of those circuits underlying those behaviors, then you've altered the probabilities. And so some of them you just may not see very much at all. Some of them you see much more than normal.
OK. And this view of motivation that I'm talking about, this hierarchy, the ultraprobability of action and so forth, has been simulated. And this an area called computational neuroethology, which is not a computational area that's pract-- you don't find much of it in this department.
But we did have it here for a while, because I had a student that was working on it. She worked with me and someone in the Media Lab who had these screen characters. Did I talk about the screen characters before? I didn't.
It's described a little bit in the book and I think I have-- let me just go to that. I mention here some earlier work in that area-- nice simulations of the cockroach, neural control of feeding.
Basically, Beer had a little robot. But he had motivation in it. So it did have endogenous initiation of behavior. And it would do searching behavior, and so forth.
And he had-- you have to have distinct appetitive and consummatory components if it is going to work. And that's what Bruce Blumberg, who when he was a student here, working on a PhD in the Media Lab-- before my student got involved, he came to my animal behavior class-- not the undergraduate class. I was teaching a grad class at that time.
And we talked extensively about how that system has to be organized in the brain, because that's what he wanted to try to understand. And then he wrote software to simulate that kind of drive, and basically developed all the background-- we would say the spinal and brain-stem levels of the control, using a large computer to handle all that background.
And then they could write programs on their desktops-- they had pretty powerful desktops-- to control the higher levels. And they simulated simple learning, what we call reinforcement-- reward-based learning. And I talked about that in the behavior class too.
And then we had a student here who took the same behavior class later, Song-Yee Yoon, who I show there. And she worked with these, you could call them affective, synthetic characters. They were screen characters that looked like cartoons on the screen as you see here. But in fact, they were robots.
They weren't programmed ahead of time. What they were going to do, what was programmed, was their drives, their sensory systems, their motor systems. Of course, they were very simplified, compared to real animals.
But the amazing part of her simulations was how human-like they behaved, even though they had no cognition, no higher cognition. They had something like a striatum, connected only to vision and audition. That was the two kind of inputs that you could give them. The visual input came from other things on the screen, part of that environment that the big computer was controlling. And they responded to us, and they responded to the other characters. OK. Yes?
AUDIENCE: So they don't make [INAUDIBLE], right?
PROFESSOR: Oh, they do. They [INAUDIBLE] because the probability of various types of behavior is controlled, remember, by the hypothalamus. And they had simulated that. And they had to have these different drives inhibit each other and so forth. All of that we talked about in behavior class.
And, of course, I pointed out to them that we don't know all the details. I can't tell you everything to simulate. You have to try it out. So what Song-Yee did was quite amazing. She could program these characters and give them different personalities by the way she set the parameters of the software.
And she did an experiment where she had students at MIT watch this for a while. She couldn't even predict what was going to happen. But she would start the simulation. The students would interact with the characters. They could put stimuli in there.
You know, they did allow the people on the outside to control and that you could even say certain things and so forth. I don't remember all the details.
But, anyway, she had them rate the personalities. She basically gave them a checklist and had them rate each of these three characters according to their personality, because we were trying to see, you know, how realistic was it to say we could control personality just by these parameters in the motivational system.
But the students made it-- it was very consistent, her results-- way, way above chance. They could consistently classify the personalities of these simulated characters, just by controlling these types of circuits that they were simulating. OK. Anyway--
AUDIENCE: Can we find this information somewhere?
PROFESSOR: We can ask Song-Yee about it. I just talked to her last week. I keep in touch with her a bit. She didn't stay in academia. She went into business, into the business world. And she went to work for SK Telecommunications Company. I think I told you about that last time.
And she developed-- she used that kind of software there to create these little avatars on their phones, so people could interact with them. She was so early, that it was very popular. But other companies, then, of course all copied it-- AT&T, the Japanese, and so forth. And they quickly made business deals with all the Japanese companies, the American companies, and basically they got sort of beat out a little bit by being so early.
And so she learned from that. And now she's working with her husband who formed this big online gaming company. He was the guy who started the online gaming where thousands of people play it at once. And she lives in the Seattle area. She has a couple of kids. But she heads up the North American operation, and her husband heads the worldwide operation.
But they are now-- more and more of this kind of software that we were just talking about is going into those games. So I think it will be exciting to see, because they don't yet have a higher level cognition.
I told her-- I gave here a copy my book-- because, of course, I didn't put these figures in the book, but I do describe it a little bit. And I suggested to her something that people haven't gone very far with simulation-- and that's the hippocampal type of learning in there also.
And that will be a major step if people can simulate that. People have tried to simulate the hippocampus, but never in screen characters like this, where you have actual behavior being controlled. So that will be exciting to see if they can do that.
AUDIENCE: [INAUDIBLE]
PROFESSOR: Yeah, I think I actually used to show it in the class. I need to see if I can find it. I don't remember now. I remember I was teaching 901 at that time, was the last-- I taught 901 until 2003. And she graduated in 2000.
AUDIENCE: Did you know [INAUDIBLE]?
PROFESSOR: So I had those few years where I was actually showing something in class. And I don't remember what happened to it. She probably has some copies of it.
I think it would be fun if I can get it from her. We could take a look. Maybe in my older versions of 901 I had actually-- I don't know what, if I transferred it all to my newer computers. It's a good question. I should look.
OK. I wanted to say a little bit about feeding and hunger. Where would you expect hunger and satiety cues to influence the CNS? Well, the obvious answer by now should be hypothalamus. It should get there in some way.
But where do the queues come from? We already mentioned the brain can detect glucose levels. There are glucoreceptors in the hypothalamus. OK.
You can link something to glucose molecules and find it in the brain. It will collect in the hypothalamus. One of the early methods was to link gold-- gold thioglucose-- OK, link gold through a sulfur molecule to the glucose. And it will collect in the brain and stay there and kill the cells.
So you basically can cause behavioral change by this chemical means. But there's much better ways to do that now. But, of course, we get many cues through pathways, through the visceral sensory system. OK.
It comes by way of the vagus nerve. For example, signals of stomach distention, which in infants-- newborns-- it's the only thing they have. They cry if their stomach's not distended with milk. And when it is, they stop crying. They're satisfied.
That, of course, develops over time into a much more sophisticated system for controlling hunger and satiety. If you don't have-- if the midbrain is the highest level, which is almost true of the newborn, but if you get animals that are anencephalic because they're born with, where the neural tube hasn't completely closed. They don't live very long, because they're born with-- you know, the whole endbrain is not fully developed. The neural tube hasn't closed.
So basically you're dealing with a newborn that the midbrain is the highest, really, functioning level of the nervous system. And they do show reflex eating, but they don't show normal hunger and satiety at all, in addition, of course, to missing a lot of other things.
So you get the reflexes. You get the consummatory patterns, very little regulation. You can always get chewing, licking, swallowing. But you get it whether they're starved or full of food. No active seeking of food. Exactly what you see in animals we talked about before-- animals where you remove the entire-- or you've disconnected the cerebral hemispheres-- including the hypothalamus.
Remember, to keep them alive, you have to leave the hypothalamus attached to the pituitary to maintain blood levels of hormones. OK. But you can disconnect that area from the midbrain.
Now we know that the mid-- well, we'll see that here-- the midbrain is capable of some of these same things. But it seems like once it's disconnected from the hypothalamus, it doesn't function well by itself.
And I think that would be different if you could wait long enough, if you keep the animals alive a very long time. That's very difficult to do. So we think core brain mechanisms are pretty critical for normal motivation in all the motivational systems.
So this is just what we've been talking about. And I mentioned the inputs to the vagus. I mentioned the blood factors. And I mentioned-- I separate here, short-term and long-term regulation.
You know, the blood factors that indicate amount of fat, for example, control the amount of eating. But that's a long-term regulator. It doesn't change fast enough to affect how long you eat. What affects that? Why do you just eat a certain amount and stop.
We're actually not like infants. We don't all eat until our stomach is distended like the newborn. Some of us may sometimes, especially at Thanksgiving, you know. But usually we don't. And it's the same for animals.
This was an early experiment of Pavlov and others, where they led the esophagus out into a bag, so the food never got to the stomach. And yet the dog would eat a meal of a certain size and stop.
It doesn't have to go to the stomach and cause stomach distension, even though there are signals from the stomach that will stop eating because of pathways going to the hypothalamus. Yet they still stop, just by signals coming from the throat and mouth.
Now, I mentioned electrically stimulated aggression. You can stimulate the hypothalamus and get an animal that acts like he's hungry. So you can stimulate in a predator lateral hypothalamus and find areas that he goes into a predator mode when you stimulate it.
So I'm saying what recently developed technique is replacing the use of electrical stimulation? Optogenetics, where you can specifically stimulate particular cells. And sometimes you can actually get them to link only to cells of a given type.
Or you can just put the virus in that puts those opsins into the cells and makes them sensitive to light. But that is now being used to study hunger, so they're getting more specific information, including information about specific pathways, although that work had gone pretty far without that technique. Now they can advance it a lot more rapidly.
Now if you stimulate attack motivation in a cat, how do you know you didn't just make him more hungry. We know the behavior is different-- feeding and predator behavior of cats. But first of all, it's important to know are you really stimulating attack behavior? In fact, there's more than one kind of attack behavior too. But let's just talk about predatory attack. It's called biting attack in the cat.
Well you have to pit things against each other. So the classic experiment-- I call it classic because I think it's so good. Maybe it's not classic because not enough people know about it. But this was work done by John Flynn and his group at Yale University, which I know a lot about, because one of his students came up to work with [? Naro ?] when I was working with [? Naro. ?] That was Carl Chi.
And he described to me these experiments in detail. They would, for example, have a cat in a room, have some mice or rats also in the room-- usually at the other end of the room. They turn the stimulus on. This is a cat who was satiated. He had eaten. OK.
And his ears would prick up. He would start looking around. And he would immediately then, after the stimulus went on, he would attack the mouse or rat.
So then they did experiments where they would put the favorite food of the cat, like tuna fish or some other fish-- cats love it. And they would leap right over that, totally ignore it to get at the mouse or the rat, indicating that it was definitely not hunger they were triggering.
They could make the animal hungry with different sites. The problem with that kind of work is that they're actually stimulating many axons. But there's always a dominant one, and that's the behavior you see.
There's obviously a lot of reciprocal inhibition of these motivational states, so they affect each other. And if the animal is hunting, he's not eating. OK. The eating is separate.
This just shows a site where they could get attack behavior. And after the experiment was over, they would make a little lesion there. Then they would let the animal live for five, six days. They just let him live long enough after the experiment was over so they could then trace degenerating axons. You have to give them a little time-- the axons time to degenerate.
And then they would trace-- that was one reason Chi came to [? Naro's ?] lab, to learn how to do this. So this experiment was done involving Carl Chi after he had worked with [? Naro. ?]
And you can see he could trace axons to other parts of the hypothalamus to almost the entire subthalamic area at this level. This is the zona incerta of the hypothalamus-- sorry, the subthalamus.
And then this is the midline and intralaminar nuclei of the thalamus-- the older structures of the thalamus-- first-to-evolve structures that connect to the striatum and also to the pallium, OK, the cortex.
And the interesting part about these pathways is neurons in here have connections to the [? neural ?] parts of the thalamus. So they're affecting-- they're sort of dating information going through the thalamus just by altering the motivational level from down here. OK.
I'm not going to go through this "drive and reward involving distinct axons." Students always find it a little difficult to understand. Read it in the book, and let me know if you can follow it. I would like to know.
Because I would at least like to start what we originally scheduled for today-- the core pathways of the limbic system. And that will lead us to studies of memory.
I want you to begin to understand why the limbic system became so critical to our memory formation, what we call episodic memory. So these are the topics we're going to talk about.
Let's at least talk a little bit about [? hypokalemic ?] cell groups before we quit. And I'll show you the [? pages ?] where you will read about it so we can go through the rest of it quickly.
Then we're going to talk a little bit about hormones. I may decide to save time and to let you just read that chapter on hormones. I can show you a few slides from it, just so you'll have a chance to ask about if you want to, because I want to spend more time talking about the hippocampus, which we do introduce today.
So two divisions in the hypothalamus. Two major divisions-- medial division and lateral division. They're very different. They look different histologically.
If you look here at a section of the rodent brain. Here you have from here to here-- the medial division. And from here to out here, the lateral division. So this is all lateral in the hypothalamus. This is medial.
Notice this very distinct cell group in the medial hypothalamus-- the ventral medial nucleus of the hypothalamus, the VMH. That is typical of the medial hypothalamus. This is from a study by Le Gros Clark.
He did this reconstruction. He used sections of the brain to discern these various cell groups. And then he reconstructed them, sort of projecting them onto the midsagittal plane.
So you can see the mammillary bodies, dorsal medial nucleus, ventral medial nucleus in human, preoptic nucleus, supraoptic nucleus. There's the big optic chiasm in human right there. And this little funnel shape. He's near the pituitary.
So if you look here in a rodent, it's the same thing, but the shape is very different. There's the chiasm up there, with the supraoptic nucleus above it. Here's the pituitary attached here-- the neural part and the glandular part.
This region where the stalk of the pituitary attaches is this region in humans, where it's called the infundibular, OK, for its cone shape. So when you see a picture like this, where these hypothalamic nuclei are, we're dealing mainly with the medial hypothalamus.
The mammillary bodies are both medial and lateral. Posterior hypothalamic areas is also broad. But it's mostly in the medial hypothalamus. OK.
So let's just do quit with this one if somebody can answer this. Information about the internal environment reaches the hypothalamus by two major means. We've already talked about them.
Now just see if you can remember that? OK. Via the-- by the bloodstream. So it can reach the hypothalamus by the bloodstream. Not just hormones, but other means too. But things coming through the blood.
And what's the other means? If it doesn't come through the blood to get to the hypothalamus, it must come from neural pathways. Afferent fibers come in mainly into the spinal cord.
We call them visceral afferent fibers, mostly through the vagus nerve. But for the head region, they come through hypoglossal nerve. There's a few even in the third nerve, but very few.
They come in through the seventh. They come in through the ninth and tenth nerves. All right. But if they come there through the blood, then we have got to understand the blood-brain barrier.
So we'll start with that next time. I'll just show you a few pictures. This is the interesting one. They inject HRP right into the bloodstream.
You know, way down in the body someplace. And they see that in most of the brain, you just see the HRP in the capillaries. It never gets out. But in certain regions, it leaks right into the tissue.
The blood-brain barrier seems to be very weak in some areas. So that's what this picture is about. There's a better one in the book, OK.