Flash and JavaScript are required for this feature.
Download the track from iTunes U or the Internet Archive.
Description: This lecture is the second of three on visual systems, focusing on retinal projections, light detection and species differences in structures.
Instructor: Gerard E. Schneider
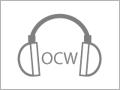
Lec 22: Visual systems, part 2
The following content is provided under a Creative Commons license. Your support will help MIT OpenCourseWare continue to offer high-quality educational resources for free. To make a donation or view additional materials from hundreds of MIT courses, visit MIT OpenCourseWare at ocw.mit.edu.
PROFESSOR: At the end of the last class, I mentioned that this invasion of the endbrain by visual pathways-- we know mostly that even though there was a direct pathway, it was very small-- the one through the geniculate body, very small early on. Major routes into the endbrain. They began at the tectum, also the pretectal area, which projected into thalamic areas, which then projected to the endbrain.
I asked there at the end of the class, what would be the adaptive advantages of that? Why would it invade the endbrain? First, I tried to give them a comical answer-- it's a way to provide better information to the striatum. Remember, visual information can reach the striatum directly from the overthalamus. Those structures weren't precisely topographic, and the striatum wasn't the structure that made really good topography as easy to form in evolution. That's my interpretation.
Obviously, that happened mostly in the cortex. In the cortex, you get this highly topographic mapping of the visual world, and those pathways came by way of the membrane and directly from the geniculate body. That enabled a lot better acuity for the learning that the endbrain was capable of, especially by means of its pathways to where? Two different places-- to the striatum for habit learning, and to the hippocampal formation, for learning about locations of the animal in their environment.
Not the locations of other animals-- the location of the animal whose brain we're talking about. The cognitive functions were that route to the hippocampus, replace information as a major part of our cognitive ability, our memory formation, but also another kind of memory-- it provided a visual route to the amygdala, which is really part of the striatum, the way I see it-- at least a caudal output for the striatum. It functions like a striatum with the learning of avoidance and approach.
Also binocular vision, which we won't talk very much about now, but that was important, too, because the neocortex made possible a lot better binocular vision by especially stereopsis. Slight difference in the visual image in the two eyes because the information from the right eye and left eye is kept separate, all the way up to the cortex, where then, you can combine that information and get depth.
At the end of that chapter 20, I mentioned-- I believe it's in chapter 20-- I mentioned some of the expansions and specializations of the visual system, mainly midbrain tectum and the thalamocortical projections of the visual system. These are the two major structures, and we're going to look at that today.
We'll be talking a lot more about transcortical pathways in the following class. I don't deal in the book with the tremendous differences among animals in retinas. They develop many retinal specialization in animals. You don't need larger eyes just to get a larger retina and get more retina ganglion cells and more acuity that way. What else do you need large eyes for? Bring in more light.
Why do you buy a camera with a big lens rather than a small lens? Brings in more light. If you want to get a camera that can take pictures in very dim light, get a camera with a very large lens opening, like F1.8 or something like that. It'll take in a lot of light. Question.
AUDIENCE: For animals, does that [INAUDIBLE] have a forward configuration in their eyes?
PROFESSOR: Excellent question. We know that animals with forward-looking eyes are usually predators and primates, and primates are usually predators, too, but not all of them. Some of them are fruit-gathering primates. But they also need good binocular vision so they have forward-facing eyes, too.
But what about these animals with the eyes like the hamster? It's got eyes that look 60 degrees out and 30 degrees in. That's the way their eyes look. That's the natural position of the eyes. I've measured it in the hamster. It's pretty similar in other little rodents. They do have an area of binocular vision. There's about at least 30-degree overlap in that central area. The center of it is 30 degrees above. They do have binocular vision, but it's pretty rudimentary compared to the primates.
Also, they don't have the high acuity that primates have. But it's still important to them. I've looked at hamster behavior a lot, and things five feet or even two feet and 30 feet away, they don't [? discern it ?] very well. But for things near them, they definitely can judge vision. They won't leap-- sometimes they do, but usually, they won't jump into places that are too dangerous because they're too high. They can see the depth well enough.
It doesn't mean stereopsis cues, though-- it could just be the various other cues, like when they move their head, the parallax that you get-- there's various cues to depth that if you study in a sensation and perception class here at MIT, you'll learn about multiple different cues for depth that don't involve stereopsis.
Now we want to look at the retinal perceptions. It's a pattern you find in all the mammals, and I should add that it's really similar in all of the vertebrates. This is the picture I showed in the last class of the cartoon of the main optic tract and the accessory optic track here. Now we want to look in a little more detail at the layout of that system-- the suprachiasmatic nucleus to the two geniculate nuclei. The pretectal region, where there's several nuclei where they terminate-- superior colliculus. I
Look at the retinal projection invertebrates, the layout of the optic tract. Look at them in several different ways of looking at it. We'll talk about species differences and relative size of structures because qualitatively, they all have the same basic layout, but there are large species differences in relative sizes as well as in the eyes are different too, so you have different levels of acuity in vision are possible in these different species.
We'll look a little bit at architectural differences, especially in the geniculate body and the optic tectum. We'll look at lamination in midbrain tectum. We'll also look at lamination in the geniculate body. And finally, we'll look at topography. I know when you first get all this, you get a little overwhelmed by all structures and everything, so I'm not asking you to memorize the topography. I will present to you in a way that does make it pretty easy to learn.
These are the questions. I put a list of questions online for you. These are things we've dealt with before, these two questions. Let's just see if any of you have an idea about it. First of all, what's the first structure reached by the axons from the retinal ganglion cells. Ganglion cells project through the nerve. We start calling it the tract when it joins with the rest of the diencephalon. What's the first structure? Exactly. Suprachiasmatic nucleus, right above the crossing of the axons. It's gotta be the first one.
AUDIENCE: [INAUDIBLE].
PROFESSOR: It's part of the hypothalamus, definitely. The area in front of that region, we call preoptic area of the hypothalamus because it's in front of the optic chiasm. Actually, we talked about the anterior hypothalamic nucleus and then the preoptic nucleus. I might apply my first statement a little bit. The forebrain subdivision you've just named, it's the hypothalamus and the major terminal nucleus in that subdivision is the suprachiasmatic nucleus.
Now remember, there's some [? store ?] over there, too, that I showed you last time. It does project the two immediately adjoining parts of the hypothalamus. We know most about that suprachiasmatic nucleus. There's very little known about the functions of the sparse projections.
People tend to think that they don't play any major roles, that's why they're so sparse. I'm not convinced of that. They could play important roles. The one thing that makes me doubt it a little bit is just that there's variability. When I study the retinal projections in a number of different hamsters, I do get differences in these very sparse projections. Some animals I see more, some I see less, but they're always there.
The next question is, what's the major difference in the nature of the projections of the dorsal thalamus on the one hand and the ventral thalamus or the epithalamus? That is, after you go past the suprachiasmatic nucleus, the axons go up the side of the diencephalon and they reach the subthalamus just above the hypothalamus.
Then they reach the dorsal thalamus, then they reach the epithalamus-- the pretectal nuclei in the epithalamus. There's a big difference, though, in the nature of the projections of the dorsal thalamus and those other groups. What is that difference?
AUDIENCE: [INAUDIBLE].
PROFESSOR: Exactly. The biggest difference is what she just said-- the dorsal thalamus and all the major cell groups-- at least the neothalamus, the more recent-- the dorsal thalamus, like the lateral geniculate body, but the same thing holds true for the ventral nucleus, medial dorsal nucleus, medial geniculate body. It projects to cortex, mainly to neocortex. It goes to the endbrain. Some neurons, some axons will have collaterals in the striatum on their way to the endbrain, but the major projection is to the endbrain.
Those other structures-- subthalamus and epithalamus-- don't project to the endbrain. There are reports that they do, but modern techniques have never been able to verify that. They don't project to neocortex. In fact, besides some ascending projections, they have a lot of descending projections. That's the major difference.
Last time, I mentioned the epithalamus as a caudalmost diencephalic segment, or neuromere. It includes cell groups for the optic tract, its dense terminations. Again, what do we call those areas of termination? We call them certain nuclei, depending on where they are. They're in front of the tectum, so we call them pretectal. They are rather different, these different groups, and they have different functions, but we lump them together because they're all in that same region called pretectal nuclei.
This is two ways to look at it. Don't worry, I'll blow this up. Here's a cross section of a level through the diencephalon. This is actually behind the optic chiasm, but you see the optic tract covering most of the diencephalon, or 'tween brain, at this level.
The pretectum up here, the dorsal thalamus with lateral geniculate is right there. Here's the subthalamus with ventral nucleus, a ventronucleic body there. There's hypothalamus. This is behind the optic chiasm. The optic tract covers all these. That's why that area is sometimes called optic thalamus, just because the axons of the optic tract cover it. These are the subdivisions written as the names for their neuromeres.
Now we know the hypothalamus is actually two or three different neuromeres. We call this the ventrothalamus, then in the adult, we call subthalamus. Then the dorsal thalamus, then the epithalamus. But note there's another way to look at the tract that I use for teaching purposes, but I think it's very useful. I just take the track all the way from the suprachiasmatic nucleus all the way to the tectum, just stretch it out. You see the retina would be way off here to the left, off the screen there to the left.
There's the suprachiasmatic nucleus. It's more commonly abbreviated SCN, and here we call it just SCH, but could note it down either way. Then I just follow the accents. I straighten out the tract. I show what happens to them. The longest ones make it to the superior colliculus where they no longer travel mainly at the surface.
The rest of the tract is mainly at the surface. There are some that travel internally. We call that the internal optic tract. But there are smaller numbers of axons that do that. The main ones travel on the surface except when they get in the sphere of the colliculus. Actually, they do travel at the surface of the colliculus early in development when they first get there, so then how do they end up down below those superficial layers? What has to happen?
They're on the surface and then they're not, further development. Either the axons that were on the surface have to die off, or cells in colliculus migrate up through them. They're still migrating when this tract is forming.
The latter interpretation appears to be true because you do see cell migrations going up to superficial gray occurring after the first axons have reached there, and when you look with stains that are capable see degeneration occurring, you don't see a lot of degeneration. We think it's actually doing cell migration.
Here you see that it looks like they're branching off. Let's do it this way. Here's that first picture again. I'm going to blow it up. But it's the same thing, and I'd point out here ventral thalamus is not the ventral nucleus of the dorsal thalamus. It's really subthalamus. The ventral nucleus is this nucleus up in here, part of the dorsal thalamus.
Here I just show the places the optic tract terminates, you see the dorsal part of the lateral geniculate body. Remember, this is a rodent. The LP is not huge, but it's the rest of the lateral thalamus. Besides the geniculate bodies, you have lateral thalamic nuclei. The medial geniculate is another lateral thalamic nucleus.
Here is a human 12 weeks post-conception. Three-month human fetus. There's the rodent, adult. Here's the human fetus. The adult human thalamus doesn't look like this at all. I just want to point out that it's really the same as the rodent. In fact, the embryo looks very similar. But then it changes. Why? Anybody figure that out? What happens to the human thalamus development after that first three months of life?
The brain of that three-month human fetus is pretty similar to a hamster just after birth. But then something continues developing in the hamster in both the hamster and human, but mostly the human after that first three months. That means, by the way, the human at three months post-conception is like an early postnatal hamster. The hamster is born in the 16th day of gestation, just to give you prompts.
But anyway, what happens? This nucleus labeled NL, the lateral nucleus, nucleus lateralus-- this is the posterior part of nucleus lateralus. That grows and grows and grows, and in primates, it really gets huge and we changed its name. We call only part of it the lateral posterior nucleus, like we call the whole thing in rodents-- the rest of it, we call the pulvinar, which means pillow. The pillow because it's bulging out so much-- because the cortex it projects to, even though it's there real early, it expands so much.
A lot of people that talk about cortex just think the sensory input comes to it and then you go from one region and the other in the cortex and then you go out through the motor cortex. Not a very good, accurate picture, even though the majority of people in this building think like that. That's not really a good picture connections of the cortex. We'll be dealing with that, including some next class, but we'll continue dealing with that with the other systems. That's what happens.
Otherwise, at this stage, these pictures are pretty similar. Remember, those divisions here happened because even a very early embryo, they show a side view with the segments. Remember the seven rhombomeres here and then a little segment we call the isthmus and then the midbrain, which is a pretty big segment, and then the epithalamus with the pretectal forming area forming here-- the venular forming here. And then dorsal thalamus, ventral thalamus or subthalamus, hypothalamus, which actually turns out to be several subdivisions.
These are the questions we want to answer now. I want you to be able to name the five main optic tract termination areas in the order they're reached by the optic tract. So here they are, they already passed the suprachiasmatic, then you have the two geniculate bodies, then they get to the pretectal area here-- I show them on this picture-- and then finally, they turn caudally and they get to the superior colliculus. Those are the areas.
One, suprachiasmatic, two, three, four, and five is for your colliculus. Then I ask what additional areas receive sparse retinal projections. Well, I already talked about some near the suprachiasmatic nucleus, but there's some other sparse projections in the hypothalamus that are someone variable from animal to animal, at least among the hamsters that I've studied.
I expect it's true of other species, too. That would be projections to the LP nucleus here. There are other little projections near the main projections that are also a little bit variable.
Then I say inputs from the right and left eyes terminate in different areas-- a separation that is especially important for creating binocular disparity cues for perceiving depth, visual objects. Describe the appearance of the distinct areas in the diencephalon of a small rodent and of a monkey. Here, we're talking about the layers in the geniculate body.
In this picture, that stretched-out optic tract, I show that. There's the suprachiasmatic. Then they reach the subthalamus. This is the lateral geniculate body ventral portion-- often abbreviated just LGV. It's also abbreviated LGNV-- lateral geniculate nucleus ventral part.
But notice here, these are axons from the contralateral eye that I'm drawing. I show a little area separated here by the dashed lines, and there at the ventral and dorsal geniculate bodies, where those accents don't go. They don't go there because they're getting input from the other eye. It's a laminated structure.
Not so obvious in the rodent, but when you go to other animals-- here's a four-layer geniculate body, which you find in some species. Then you find that the contralateral eye is projecting to the outermost, the layer nearest the optic tract, then it skips a layer, then it projects to the next layer, and then to the last layer. It projects to one and three, not two and four.
In primates, like monkeys-- not all primates, but at least monkeys and apes and humans-- you have six layers. Sometimes you see seven in some parts of the geniculate body, but usually, we number six, so we'll see that. Then the axons go through and over the LP, the lateral posterior nucleus. It's the rest of the lateral thalamus, the part that grows so big in humans.
I show a few terminations there. It's somewhat variable, but they are there. They're sparse terminations. Again, we don't know what those do. Maybe we don't need to know because those stained neurons where those sparse projections occur get very heavy visual input, but coming from the colliculus, not directly from the retina.
One of the ideas about how the geniculate body appeared was just that-- these sparse, scattered projections that if some reduction of the colliculus occurred, they just sprouted more there and that was adapted. They have a shorter route to the cortex, so the geniculate body evolved. Be that as it may be, the next structure, then, is the pretectal area, and then finally, superior colliculus. Or optic tectum, but for mammals, we usually use the term superior colliculus.
Let's look at the geniculate body now. We look at animals with this kind of layer, but even more. Here's the geniculate body of a monkey. It's similar to a color picture I took from David Hubel from a book that he has online that anybody can download. Very nice book.
Unfortunately, David died just recently, but he was very productive visual neuroscientist who did many studies of both cat and monkey geniculate striate system, looking mostly in the visual cortex. He did the work with Torsten Wiesel, who retired now but has had a position at Rockefeller University for the last quite a few years after he left Harvard from his work with Hubel.
Here, it shows you how the number-- oh yeah, where did this horseshoe shape come from? That's not what I'm showing here. Can you figure that out? Let's go back to the embryo. It looked like this. Monkey is the same. This structure here grew and grew and grew, so this structure got pushed this way.
The pulvinar, in growing so big, the thalamus in front of it also grew and so this thing that's on the side of the thalamus here, side of the thalamus got pushed over like that and also got rotated so the geniculate body ends up on the back of the thalamus. It goes that way and this becomes like that.
And the optic tract, which was out here, is now under here. That means this is the optic tract surface. Those are the axons. The tissue is thrown off here. But that's where the optic tract is. Topologically, it's the same as this picture here.
You see the way they number them-- they always start numbering them nearest the optic tract. One, two, notice the first two layers are larger cells and then the smaller cell layers, or parval cellular layers, are three, four, five, and six. Again, you have half of them that are contralateral input and the other half are getting ipsilateral. Almost always, the contralateral is projecting to surface layer and to the last layer here and to one of these middle layers. It varies a little bit among species.
Here's a human. This is a human, a picture that was donated to me by a former student who developed a silver stain that stains cell bodies. It gives you a very high contrast picture. This happens to be from a human case who had a pathology of one eye, so the cells of the of the eye that gets ipsilateral projections, the cells have shriveled a bit. This is one of the magnocellular layers here, and here's the other contralateral layer and the last contralateral layers. These are the layers getting ipsilateral projections.
Most of the other magnocellular layer, we don't see here. All of these cells here are all parts of that lateral thalamus, the pulvinar nucleus. There's a small part of it that they call-- the part that's inferior pulvinar-- it's similar to LP of the rodent. Often they do name an LP, too, but the homology is not always that clear.
This is a picture from a very famous neuroanatomist in the early part of the 20th century, Le Gros Clark, who didn't have access to beautiful optics for taking low-power pictures and all that, so he drew them. He drew these beautiful pictures, in this case of many different primates. At that time, they thought the treeshrew might be the most primitive primate. It turns out to be similar to very primitive primates, but it's actually an insectovore.
You see the different patterns of lamination that occur in these different animals. They don't all have identical structures. Binocular separation-- the anatomy underlying that binocular separation of the brain has evolved differently in different animals. In these pictures, the old world monkey, the guenon, is most similar to the human.
This picture here is fairly close and level to that picture of the photograph. Now I want to go to the rodent, which are usually used in laboratory work-- a lot of the studies of many systems. I use side views and I show-- here's a very simplified picture of it.
The outline here is a fairly accurate view, a side view, of the upper brainstem of a hamster. You see the inferior colliculus and super colliculus of the midbrain here. This is our thalamus. The dorsal thalamus would be all through here, but the geniculate body here, way out at the edge, here's the two parts-- dorsal and dentral nuclei.
Remember, I said that the ventral nucleus and lateral geniculate is really subthalamus. Remember, it's a curved structure, so this is at the lateral edge so it's much higher up. As we went deeper into the thalamus, it would be way down here.
What I show here is a little schematic of one little bundle of optic tract axons coming from the chiasm up the side. I don't show the terminations in the suprachiasmatic nucleus because those are different axons, but I show that axons that go to the geniculate body are usually branches of optic tract axons that go further. They go to the tectum, and often the pretectum, as well.
I also show where the major input to the LP that provides the LP with visual information doesn't come from the retina even though there are a few projections there. They come from the superficial or visual layers of the superior colliculus. It projects to both the LP and to the outer layer of the ventral nucleus of the lateral geniculate body.
Here's the question that I want to answer next-- there are axons that leave the main optic tract and terminate in the small groups. There's up to three of them. They're described as what kind of optic tract? The word is accessory optic tract. This is an anatomical reconstruction of the hamster where I have labeled the axons, all of the axons that I could, which is most of them.
I get the entire optic tract. This is done from serial sections, so the sections were actually like this but pretty close together. I then reconstructed the entire optic tract from chiasm to colliculus.
In this particular brain, I was using stains for degeneration. I didn't get a good picture of the suprachiasmatic nucleus, so like most scientists, I never lie with the data I present-- I don't show it. But in fact, with other techniques, I could see it, and it's right here.
What I'm showing here is the accessory optic tract axons, and you can see why they're called accessory optic tract. They need that main track that goes all the way from chiasm to colliculus here. Here are some that leave just below the cerebral peduncle and they travel caudally and they terminate medial to the peduncle, right there where the nucleus sort of hugs the medial side of the substantia nigra in the cerebral peduncle.
Others just travel caudally below the thalamus here. They just sort of peel off at the level of the ventral lateral geniculate body. They terminate in a little nucleus here. Some axons go down this way and get to this other one. There's even some axons growing in another direction. That's called the transpeduncular tract.
Finally, here's a little tract that goes from the pretectal area. It leaves the main tract, seems to go down to the lateral [? termonucleus, ?] but it has another little nucleus there way out at the lateral edge of the pretectal area. That's the accessory optic tract-- a very interesting system because all the cells in that tract respond to movement of the whole scene across the retina. No matter where you are in the retina, the cells will detect movement in the same direction.
When does that happen? When the whole animal starts to fall? It happens when the animal is locomoting and so there's straining of the virtual world past his eyes. It does the same kind of functions that the vestibular system does. It gives visual signals to indicate changes in head direction and eye direction. Very important for locomotion and very important if you need to keep track of head position.
Then I just want to show you what that reconstruction is doing by taking photographs of a similar brain. Here, I didn't label it. Here, I did. Here, because of the way I've shaped the light, you can see major tracts because they're wider. That's the lateral olfactory tract. There's the optic tract. There is a pathway carrying auditory input from hindbrain up to the inferior colliculus.
There's the bundle-- you see these two bundles here? We cut those when we remove the cerebellum. Those are the cerebellar peduncles. There's hypothalamus down below. When I removed the brain, I tore the pituitary off right there. That would be the mammillary bodies at the caudal end of a hypothalamus.
Here, I've labeled them and I've labeled a few other structures. This structure here is not thalamus, it's part of the endbrain-- it's the corpus striatum. I show you exactly where the geniculate body is.
Now you say, well, what do you mean exactly? Because I can see the shadows there. I can see them there. I know that that's exactly the edge of the dorsal nucleus, and this is the ventral nucleus right below it. There's the optic tract, hypothalamus.
This big bundle here that emerges from behind the optic tract-- those axons coursing through the corpus striatum here, but in the rodent, they're all separated. There's a bunch of little separate bundles that collect and form the peduncle. It comes along the side of the diencephalon and passes right on into the Pontine region here.
When we study the auditory system, we'll learn that this is called the lateral lemniscus. That bump right there behind the cerebellar peduncles, that's the cochlear nucleus. There's the stump of the eighth node right there.
One way to learn this stuff is to study reconstructions like this, look at these photographs and try to learn to pick out the structures. Right now, this is midbrain-- you should know what the colliculi are. You should know the optic tract and the hypothalamus. Here, if I've already told you, I've removed the hemispheres and the cerebellum.
This has got to be [INAUDIBLE] hemispheres, and there's only one big structure like that-- it's corpus striatum. One way is to cover those up and in the book, it's done so you can just put a piece of paper and still see the lines for the labels. I want you to do that in your book. Cover up the labels and see if you can learn them. Learn what these structures are on this kind of picture.
This kind of picture and this kind of reconstruction were very important to me for a lot of experiments I did because I needed to do neurosurgery where I would open up the brain. Of course, I would only see a small part, but I learned the landmarks so well that I could open it up and also using blood vessels, I could see these structures and see the boundaries, that way I could make injections or even lesions in small regions.
This is another, somewhat easier view where I have the adult brain on the left and the brain of a newborn on the right. Here, I just labelled major parts of the brain-- the olfactory bulb, neocortex the superior colliculi, inferior colliculi, the cerebellum, and the medulla oblongata, the caudal part of the hindbrain.
The [? lateral ?] part of the hindbrain is underneath the cerebellum, and the cerebellum is part of it. What I want you to know here-- look at the cerebellum here in the baby. There's just this tiny little collar behind the inferior colliculus. It's mainly developing with huge numbers of cell migrations from rhombic lip of the hindbrain-- just developing in the newborn hamster. It's born, remember, at a stage where it's like a two-and-a-half- to three-month human fetus, which means that a two-and-a-half month human fetus looks just like this, too. The cerebellum grows mostly after that time.
One way to study that-- use the book and make yourself a card and cover those up and make sure you know what all those pointers are pointing towards. This one should be the easiest, this one will be a little harder.
Can somebody tell me what E is? Optic tract. What about D? Hypothalamus. Did anybody do C?
AUDIENCE: [INAUDIBLE].
PROFESSOR: Head, right. Cerebral peduncle. The abbreviation for cerebral peduncle is PED. I didn't label the pons, but this would be the pons right there. This is the trapezoid body, which I don't name, cochlear nucleus, cerebellar peduncles. Lateral lemniscus. I just labelled the ones here that-- we might put a diagram like this.
We wouldn't put all those things, but we might ask you to do a-- give you a bunch of terms and say, well, which one goes with each of these letters? So if you're at least familiar with it, you would be able to do that. Here, the same thing. Here, I've covered up. Just name them out for me. What's that?
AUDIENCE: [INAUDIBLE].
PROFESSOR: What's this?
AUDIENCE: [INAUDIBLE].
PROFESSOR: OK, or cerebral hemisphere. Either one would be proper. What's that?
AUDIENCE: [INAUDIBLE].
PROFESSOR: OK. Notice most of it is exposed here. Here only part of it is exposed. In most animals with even bigger hemispheres, you don't even see the membrane from a dorsal view like that. OK, and this one?
AUDIENCE: Cerebellum.
PROFESSOR: Cerebellum. This? Caudal hindbrain or medulla oblongata. I'm asking you another question here about naming these structures. I said, what would make this more difficult during a neurosurgical procedure? Think about it. In a neurosurgical procedure, you don't expose such huge amount of brain. You want to make as small window as possible so you don't damage so much tissue. That's the first problem. The second thing is there's a lot of blood vessels.
In fact, you have to be very careful not to go through any really big ones because you'll get so much bleeding that you'll be spending all your time stopping the bleeding, and you do spend a lot of time doing that in neurosurgery, although now we have a method to apply that does stop the bleeding of all those smaller vessels, just not the huge ones.
Look at these other pictures for next time and just see if you can figure out what's exposed. I use different lighting. This is the same kind of brain stem that you see here with the hemispheres removed. You can see various structures. Here I've done a little more removal up front here.
See if you can figure out what those things are. I do name lot of them in the book. I don't think I show all these pictures in the book. I wanted you to see how if you adjust the light a little bit, you can actually see boundaries. Look at this one. There's the superior colliculus. There's the pretectem. Look at this one, even clearer. There's the boundary between the superior colliculus and pretectum. Look at how clear this boundary is. And this one. Pretectal area, LLP, geniculate body.
That is the bundle carrying information from the amygdala, which we will study soon. This just shows what it looks like in the embryo when the axons have first grown back to the tectum-- a very straight pathway back to the tectum. Next time, we will start in this area and talk about the midbrain a little more, just look at these pictures. They're in the book. I'll give you a chance to ask about them, and we'll go through some of these before we go on to the endbrain.