Flash and JavaScript are required for this feature.
Download the track from iTunes U or the Internet Archive.
Description: This lecture discusses the differentiation of the brain vesicles, including developmental distortions, the midbrain and the forebrain.
Instructor: Gerard E. Schneider
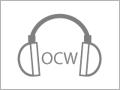
Lec 11: Differentiation of ...
The following content is provided under a Creative Commons license. Your support will help MIT OpenCourseWare continue to offer high-quality educational resources for free. To make a donation or view additional materials from hundreds of MIT courses, visit MIT OpenCourseWare at ocw.mit.edu.
PROFESSOR: A little more about the hindbrain. I want to talk about the changes that sort of distorted that simple picture of the embryonic hindbrain. We know it becomes a lot more complicated looking in the adult, but there's also this idea of changes in relative size of parts. And more than that, there are migrations of cells from the other plate that form the cerebellum and really distort things.
And we talked before about some of these changes that you see in specialized creatures, like these fish specialized for taste like the buffalo fish and the catfish, the catfish that tastes with his whole body. And then the huge cerebellum develops in some of the electric fish, animals with electroreception.
But we want to talk now about cerebellum. So first of all, what's the meaning of the word pons? And what's the major input and output of the pons? Where is it? In the rostral hindbrain, what part?
Remember what pons means? It means bridge. Why do they call it a bridge? Well, if you look at the brain from the ventral side, it's a really obvious structure. It's wider than the rest of the hindbrain. It's way up at the top of the rostral part of the hindbrain. It's white because of myelinated fibers.
What are all those fibers doing? There's a lot of cells there, and there's a lot of fibers. The cells we call the pontine gray, the pontine gray matter. And it projects to what? The cerebellum.
So it gets very big in animals with a big cerebellum. Well, what is its major input? I showed it once in this picture. This is a picture that was originally of the primitive brain, and then I added the neocortex to it to show some of the long pathways going up this neocortex, and then in this one coming down from neocortex.
And I'm showing axons here that generally go on into the spinal cord, but they have collaterals that terminate there in the pons. And you can see here what the output of the pons is. It goes to the cerebellar cortex.
So that's the answer to number 16. If you wanted to give a general answer to that next question, what causes quantitative distortions of the basic structural layout of the hindbrain? What's the major distortion that occurs in the development of the hindbrain of humans and other primates? And that's mainly what I want to talk about here.
The distortion has to do with the size of structures. Just like you saw the hindbrain distortions caused by these fish with a very specialized taste input. What were the cell groups that grew so much, became so big and distorted the brain stem in those animals like this? That's a hindbrain distortion, yet it's become the biggest part of the brain.
What's the cell group? What kind of neuron are they? Sorry?
AUDIENCE: Gustatory.
PROFESSOR: Gustatory, yes-- taste. They have specialized taste, so a lot of them must be secondary sensory cells representing taste. Now, there are other cells in that lobe too, because they actually in their specialized taste organ in the palate, way in the back of the throat, they also have some contractile organs there, so that it has a motor component that's included in that huge lobe.
OK. So why is that the cerebellum gets so large in the primates? It has to do with a very large neocortex. The neocortex seems to need a cerebellum to coordinate its output.
For one thing, output isn't controlled just by neocortex. It's controlled by sending inputs that also go the cerebellum-- mainly proprioceptive, but also somatosensory and visual, even some auditory input. So all these different senses with slightly different timing getting there have to be adjusted and coordinated so they can get to the output at the right time, and that's what the cerebellum is largely about.
That's not what most neuroscience classes will teach you, but that's what I'm teaching. I have to see why that appeared, why it was so important throughout development. Yeah?
AUDIENCE: [INAUDIBLE].
PROFESSOR: That's a good question. Is there a correlation between proprioceptive input-- you mean in quantitative terms, the amount of proprioception, the amount of it? Well, you could look at vestibular input as a kind of proprioception. It's affected by our movements all the time. It's giving us feedback on our movement, just like feedback from the joints, feedback from the muscles. They all give feedback, monitoring our movements.
So I would expect some correlation. But it's interesting that the cerebellum develops very early, and it develops as basically a vestibular organ. And then later it acquires all these other inputs. As they expanded, they all seem to want to get to the cerebellum as well as into the forebrain.
Then I introduce another term in question 18 here. What is the role of the rhombic lip? It's a structure seen during the development of the rostral hindbrain. You remember what the rhombic lip is? Did you read the chapter?
I realize you're getting some of these concepts for the first time. We're going to talk a lot more about development in a couple of the later sections of the class. One coming up pretty soon, where we'll talk about axon growth. But here we're talking about-- and we'll talk about this actually in the very next class when we talk about forebrain.
So what is the rhombic lip? It's a transient structure. There are very large numbers of neuroblasts there that migrate from that region into the cerebellum, into the roof plate of the hindbrain, forming the cerebellum at the rostral end of the hindbrain. But they also migrate from there to other positions in the hindbrain. They form the structures that project to the cerebellar cortex. Those structures all seem to arise in the rhombic lip.
Basically, the rhombic lip is in the [INAUDIBLE] plate, cells migrate from that region, and that's what leads to these distortions. They migrate into the cerebellum. They migrate into cell groups.
This is where it is. This is where the cerebellum forms. And the rhombic lip is huge numbers of neuroblasts that form [INAUDIBLE] here. And that's what leads to the formation of the cerebellum.
And here, if you look at this picture where I've sort of depicted-- I don't have an animation, so you've got to use your imagination. Here's the original embryonic rostral hindbrain. And there with the red arrows I'm showing migration cells from the rhombic lip into the roof plate, so those are going to form the cerebellum itself. But they also migrate down to form the pons. And then some of them migrate more caudally and form the inferior olive, another major source of input. These are really the two major sources of input from the hindbrain into the cerebellum, from the pons and from the inferior olive.
And then as these migrations occur, as the cerebellar cortex grows, you can see in relative terms, it gets very, very large. I've not even shown its full size in a human. And it grows on the ventral side too as the pons forms. And here I'm just showing that the projections from the pontine cells are decussating. They cross there within the pons and go to the cerebellum. on the opposite side.
You say, well, why did they have to cross? Remember, we talked about decussations and how the sensory world comes to be represented on the opposite side in the midbrain and forebrain. But this is hindbrain. So why are they crossing?
Well, that projection from the neocortex is not a cross projection. So the right hemisphere projects to the right side of the pons. The right side of the midbrain tectum, for example, projects to the right side of the pons. But the cerebellum is not a crossed system. So they'd have to cross there in order to match the inputs they're getting from the spinal cord and more caudal hindbrain.
This is a picture of a human rostral hindbrain. So here's the original cross-section, and look what's happened to the roof plate. Here, you're just seeing the deepest part. There's only a little bit of cortex even showing there. It would go way out to here someplace and way up above the seal, if you saw the whole thing.
What you're seeing there is the output structures of the cerebellum. These are called the deep nuclei. The one that looks like it's corrugated is the dentate nucleus. The animals with the largest cerebral hemispheres have the largest dentate nucleus. So it's very large in humans.
And then there's an intermediate one, the emboliform nucleus. And then medial ones here, the fastigial nucleus, more concerned with axial muscle control. And the most primitive parts of the cerebellum are those, this medial area here.
OK. So all of this is pontine gray, the light areas, because this is a fiber stain. And then the corticospinal tract, which is at the base of the midbrain, it penetrates right through the middle of the pons. So here you see those axons, the corticospinal tract axons, cut in cross-section. They form a more compact bundle, again, at the very base of the hindbrain. They form the pyramidal tract there. So we'll come back to that again in a little bit when we talk about the motor system.
Right now, we want to talk about the midbrain. This question, why a midbrain, occurred to me when I thought about traditional neuroanatomy textbooks and the way they treat the midbrain. It generally is given pretty short shrift. They treat it as basically a reflex center, controlling eye movements, the pupillary light reflex, auditory reflexes, and it serves as a relay of auditory information to the forebrain.
And it serves as a very important visual center in primitive animals. But there's been enough studies now of the membrane and especially motor control that we can say a lot more about it now than the information that led to those medical school traditions.
So we get a good idea about the evolution of the midbrain and forebrain from animals resembling the most primitive chordates that we discussed earlier. And of course we get many ideas from comparative studies of vertebrates. We don't get much information from skulls because the midbrain is-- primitive skulls, of course, can tell us something because they will be the shape of the midbrain, will be visible in some skills, where the forebrain is not so huge.
So these are my initial answers to why we have a midbrain as well as the forebrain. So I say the midbrain together with early components of the forebrain, there's a kind of rostral extension of the hindbrain that enabled visual and olfactory control of the motor patterns, because olfaction develops at the very rostral end. That was the reason the endbrain started to form-- the olfactory input there. Otherwise, think of amphioxus before-- it probably doesn't even have olfaction. But it's got a forebrain, and it's mainly just a diencephalon with some secretory cells, important in endocrine control and probably control of motivation, just like it remains important in vertebrates.
But for vision-- and vision comes in. Where? Even amphioxus has the pigmented cells right here. We already said it was diencephalon. That's where the visual inputs come in.
OK, so for vision and olfaction ought to affect movement, they formed links in the midbrain. Generally, when connections first evolve, they're not long connections, because connections start out from structures that are small. Then, as they grow larger, the largest structures become more connected. There's a general rule in comparative anatomy-- larger is more connected.
So we have these huge cerebral hemispheres. It's got connections to all parts of the spinal cord, hindbrain, midbrain, diencephalon, as well as many connections within the hemisphere. But animals who are very small, cerebral hemispheres do not have these long connections.
They also added controlled by motivational states, and we will see, the midbrain can include structures that are involved in motivational control. It's not only the hypothalamus that does it. Remember, we talked about the hierarchy of control, or autonomic functions like temperature. So it's not just the hypothalamus that does that. The midbrain does it, the hindbrain does it, the spinal cord does it. So we'll look at that in the midbrain.
And then this is just about those structures. There had to be visual and olfactory pathways from those systems, from the tweenbrain and endbrain, as well of inputs, of course, from more caudal structures, including the cerebellum that come into the midbrain.
So first of all, what are the two inputs carrying information about light levels into the central nervous system? So we're going to talk a little bit about primitive vision here, and then about primitive olfaction. So for vision, what are the two inputs?
Lateral eyes, that's one of them. What's the other one? What's it called-- the eye on top of the head. Yeah, you don't have it, I know. The pineal eye. It's an actual eye in many creatures. Many amphibians have a pineal eye.
An early role of that optic input-- no doubt, before even their image forming eyes were present, just detection of light levels-- was control of the rhythm of activity, which was different in day and night, because it made a big difference in efficiency. It made a big difference in survival of the animals. They were active during certain periods of the day.
And we know now that cells developed there that had an endogenous rhythm, a circadian rhythm, but it was affected by light levels. They were active either during the day or during the night. So here are the two inputs, pineal eye and retinal input, from lateral eyes coming right into the hypothalamus, the most primitive visual connection.
And in that hypothalamus that we see those two visual inputs, there was mechanisms for controlling the sleep-waking cycle, altering physiology and behavior, involved both the epithalamus or the pineal eye connected, and the anterior parts of the hypothalamus. And in a modern mammal, if you damage that anterior part of the hypothalamus, as Nauta did many years ago-- before he even was a neuroanatomist and doing a PhD thesis, he made lesions in hypothalamus, because he studied and he realized, these must be crucial for important controls of the animal's life. And he found major centers for controlling wakefulness and sleep. He's still cited for those findings.
But other, we could say, the cyclical motivational states are influenced by this biological clock. They're regulated by mostly the hypothalamus. Cyclical forging, feeding, drinking, nesting, and other things. But in order for the visual inputs, we just deal with vision now. In order for those inputs to control local mode responses more directly-- that is, not just through controlling the circadian rhythm-- then they had to have links to the midbrain.
Now, when it comes to olfaction, it was and remains an important control of behavioral state. And these are the two main things olfaction did for animals-- learning object identities, remembering them, altering behavior according to what those objects were, so they could detect sexual identity and individual differences. They could discriminate what was good to eat and what was bad to eat-- they learned that just through olfaction.
Why was it so important to do it through olfaction and not just with taste? Well, to identify food, if you had to taste it, well, you'd have to come right up and touch it. But with olfaction, you might be able to do it at a distance, so there was a big advantage-- no doubt promoted the evolution of the olfaction, olfactory system. For the olfaction to control behavior on the basis of those object identifications, you needed pathways going down to the motor system.
And I point that out here at the bottom, and I point out the structures that were involved. We call them ventral striatum and the amygdala, are the precursors to those structures. Their main outputs were to the hypothalamus. But the hypothalamus likewise, to influence movement, most of the pathways went through the midbrain.
And then, of course, it was important for detecting places too-- good places, bad places. They had to learn them, remember them, direct their behavior, according to whether they were something desirable or not. And that led to the evolution of what we call the medial pallium, which became the hippocampus in mammals. Outputs went through the ventral striatum and hypothalamus, and then of course to the midbrain.
And this just expands on that a little bit. I talk about the approach avoidance movements that involves both of these kinds of control. And so for odors to influence locomotion, the projections went to specific places in the hypothalamus and the midbrain that had been studied, especially by the electrophysiologist, and I'm going to identify those areas for you in a minute here, and we'll come back to it when we start talking about the motor system, locomotor control.
So escape from predator threat we've talked about before. Now we'll see what that's led to in locomotor areas of the brain. And then the other function for controlling orienting, how is olfaction involved in orienting? Olfaction doesn't really give you a sense of place except if you're near a good place or near a bad place and they smell different.
It certainly will affect the motivation of the animal. And that will affect the response of the animal to visual, auditory, and somatosensory inputs. So that's how it affects orienting. You can trace those pathways.
So here's the midbrain. This is the structure we're talking about. And now some more specific questions-- what are the three major types of multipurpose movements controlled by descending pathways that originate in the midbrain? Multipurpose movements you have to separate from specialized movements.
Give me an example of each. A multipurpose movement is used in various motivational states. We use it when we want food. We use it when we want sex. We use it when we want to do this or that or the other thing. There are certain kinds of movements that are used for many different purposes.
There's other movements that are very specialized-- courtship movements, for example, sexual movements. They're very particular. And there are many movements like that. In an evolution, fixed action patterns, meaning genetically controlled patterns of behavior, evolve both types, multipurpose and special purpose.
So there's three major types of multipurpose movements controlled by the midbrain and pathways from it. We want to know what the structures in the midbrain are that gave rise to those pathways. Can anybody name one of them, either the structure or the type of movement?
Orienting, turning movements-- that's a general purpose movement. We use it for all kinds of behaviors. We need to be able to orient our senses, orient our heads and eyes towards something. That's one of the general purpose movements.
And what's the structure so important for controlling that? You say, well, the visual cortex. Well, that came much later. Let's talk about the midbrain. What was the midbrain structure so important in orienting? And most animals, even if they have a large cortex, still use that structure. The pathway simply goes from the cortex down to the structure to control orienting.
It's the surface of the superior colliculus, or midbrain tectum. We saw a picture a while back of a predatory fish with enormous optic tectum. Remember the barracuda I showed you? Enormous tectum, bigger than any structure in the brain.
Well, we're very visual animals too. Why isn't it so big in us in relative terms? Because some animals, the types of orienting they do are much more under the control of learning. And the midbrain is only good for very short-term kinds of learning. And we'll talk about that again in a minute.
I want you to be able to name two pathways that originate in the midbrain and descend to the spinal cord. Why aren't there three? Because the third one we don't know as much about anatomically. We know about it from physiology. It's some very nice physiology.
And I use the physiology data as well as anatomical data in coming to these descriptions. So here's a typical picture of a midbrain of an animal like a rat or a mouse or a hamster. When I first drew these, I drew them from memory. And the animal I'd use the most was the hamster. I had also done work with rats and mice and a number of other animals.
So here is that optic tectum, except we usually call it the superior colliculus in a mammal. We think of it as a visual structure, but the visual layers are just these superficial layers. What are the axons down below? Well, it must be the input coming in from the eye, the retina, and from posterior visual areas of the cortex.
This is all superior colliculus down here too. You don't need that much colliculus just to get output to the lower brain stem and to the upper spinal cord to control head and eye movements. Other sensory inputs come in there too, and they tend to match the visual inputs. They match them in representing the same parts of space. So auditory and somatosensory inputs come out of here.
If I am working with a rat, say, and I'm looking at the part of that optic layer that's responding to this visual field right out here-- remember, the rat's eyes are looking up here this way, 60 degrees out, 30 degrees up, at least for the hamster, very similar for the mouse. So here's the two eyes looking up. And I show him something up here, and I get a response from the colliculus in the upper temporal field, somewhere in here, fairly far back from the tectum. Now, if I come close to him, what am I going to do? I'm going to touch whiskers here.
If I stimulate those whiskers, this activates the same region but in the deeper layers. And in the middle, there's auditory input. And the auditory input in many animals also gives you a pretty good map of space. Like the cat, the owl-- very, very good at auditory localization too. The owl can hear rustling on the forest floor below his tree and know where that mouse is even though he never sees it. He just hears the rustling. And the cat is pretty similar in localized sounds really well.
I'll try to go a little faster here. So the three output systems, I list them structurally here, functionally here. Descending axons from the midbrain locomotor area is the first output system. It controls locomotion involved in approach, avoidance, in exploring, foraging, seeking behavior or various types. It's a general purpose movement. That's the axon system, studied physiologically in some beautiful experiments by a number of different investigators.
Then the tectospinal tract from the deep layers of the superior colliculus or optic tectum. It's controlling orienting movements-- that means turning of the eyes and head. And finally, rubrospinal tract. Rubro in Latin means red. So rubrospinal means a pathway from the red nucleus to the spinal cord, and that's controlling limb movements involved in exploring or reaching and grasping. Those are the three multipurpose movements we're talking about-- locomotion, orienting, and we'll just use that term, grasping. We'll come back to those again when we talk about motor system.
Now, this is from a physiological study using electrical stimulation to define locomotor areas in the brain. This was done in the cat by the Russian, Orlovsky, who did some very nice work on this. And what I did is I traced two of his drawings where he was showing the stimulation sites. And he outlined the most areas where he most easily elicited locomotion from the hypothalamus, and that was called the hypothalamic locomotor area.
There is a nearby area in the subthalamus where you can also get locomotion. People talk much less about it, but it's there. And here is the area in the caudal midbrain. Now, this is the sagittal section. So there's thalamus. There's the inferior colliculus, the caudal part of the colliculus. And that's the region. It centers in an area just in the reticular formation below the inferior colliculus.
And here it is in a section. This is a section at this level. There's the inferior colliculus. That means lateral lemniscus, which we haven't talked about yet. It's an auditory pathway carrying auditory information from the hypothalamus up to the inferior colliculus. But there's the area centered in this area right below the inferior colliculus-- it's part of the reticular formation-- that when you stimulate you get locomotion. Sorry?
AUDIENCE: [INAUDIBLE].
PROFESSOR: Yes, it does get input. If we just draw-- here are some major pathways-- I'll just sketch them here-- from the hypothalamic locomotor area to the midbrain locomotor area, from superior colliculus to the midbrain locomotor area. And from the striatum way up here, there are also pathways that go to that structure. Remember how I talked about how olfaction can affect locomotion, well, that's how it does it-- through the striatum. If you want, I'll put another slide in and draw those back when I put this online.
All right, I just defined the terms because some people get very frustrated when I use abbreviations, and they don't know what they mean. But in fact, you can understand the basic thing here without knowing what all these things are. It doesn't hurt to read them, because the more you encounter them, the more likely you are to remember something later on when we study them more specifically.
We didn't talk about the pons. There it is in the car. There it is in the cross-section. So this is actually rostral hypothalamus down here. That's all midbrain up there.
This is just what we talked about. Now about the other two types of movement-- orienting and reaching and grasping. Here are the pathways for orienting. This is an output cell from the superior colliculus. It's a very large cell. There's many of them in the intermediate and deep layers here. And here, the axon crosses over to this position and descends to the caudal hypothalamus and to the rostral spinal cord, the cervical spinal cord.
And here is a cell in the caudal part of the red nucleus, the output part of the red nucleus-- large cells that send their axon across the midline, they go to a more lateral position, and as they descend, they go more and more lateral. So in the hindbrain, they're way out at the lateral edge.
From the red nucleus, descends to where, if it's going to control this kind of movement? Where does it have to go? From the red nucleus to spinal cord enlargements, where the neurons are controlling those movements. Movements of the what, too? To the spinal enlargements.
So I'm not showing the locomotor area there. That's defined just physiologically. And then I have a slide just showing all the sensory systems in or passing through the midbrain. So visual inputs up here. Auditory inputs coming from inferior colliculus at this level are just seen out here. They're on their way to the thalamus.
The somatosensory inputs are passing through, so the spinothalamic tract also goes to the tectum there. These are the medial lemniscus and trigeminal lemniscus. They'll stay in approximately that position. They'll enter the caudal thalamus and terminate in the ventral part of the caudal thalamus.
Visceral inputs would be up here. And then the pathways descending from the spinal cord are in blue there. That's the cerebral peduncle. I name it here, cerebral peduncle. They're fibers from the cortex. They're going not only to the spinal cord and hindbrain but also to the pons.
So we talked about this before, the roof of the midbrain with these little hills, the colliculi. I said, it shows great variations in size, remember? Here was the picture. If animals are more specialized for using audition, they tend to have very large inferior colliculi, like in the bat and the dolphin here, the echolocating bat. Whereas if they're more specialized for especially innate visual reactions, like in the wild goat or the prosimian primate, the tarsier, then the optic tectum is larger.
And as I mentioned, some animals are specialized in vision. We've gone huge visual cortex, as in the higher primates. The optic tectum is often well developed in these primates too. But in some, like humans, we're so dominated by learned visual reactions that the colliculus is still very important, but it is not as expanded as it is in these animals.
OK. I'm going to just show you this very quickly. I'd like you to read it. I divide the midbrain into two main regions. First of all, what are the limbic midbrain regions? The central gray and the ventral tegmental area.
If you stimulate these areas in the central gray, you can get a lot of different behaviors. But it's dominated by behavior that indicates they don't like being stimulated there. Quite a bit of the central gray is involved in responses to pain-- not only, though. There's other functional areas in that, just like there is in the hypothalamus. There's an area concerned with sexual behavior, there's areas concerned with temperature regulation, and so forth. But a lot of it will give you, they'll react like they're in pain.
Whereas down here, it's the opposite. They like to be stimulated down there. So if they respond to taste, if it's a very good taste, taste system projects directly into that area. Again, an animal with a very large cortex, more of the input comes from above, but there are direct projections from the taste nucleus there. Very bad tastes, though, are going to reach the central gray more.
OK. You can divide the entire midbrain into these two systems. Those two structures I just talked about we say are limbic related areas, because they get heavy connections from the fringes of the hemisphere, the limbic areas related to hypothalamus. Remember, I defined it that way before.
So these are the areas related to the hypothalamus. The somatic areas are all the other areas. And here they are, colored for you. The limbic areas, central gray and ventral tegmental area-- all the rest are somatic.
Except down here, we look at the substantia nigra. I've shown that the medial area is really limbic. It should be lumped together with the ventral tegmental area in its connections. And if you follow these regions forward into the tweenbrain, you see that the limbic areas are interconnected with the epithalamus-- that's where the pineal eye comes in-- and the hypothalamus, whereas the other regions, if you follow them forward, you're in the thalamus and subthalamus. Those are the two regions.
So we'll deal with these questions a little bit next time and just review some of these connections, talk about the variations across species. And that's it. This is just a summary of all the long connections going through.