Flash and JavaScript are required for this feature.
Download the video from iTunes U or the Internet Archive.
Description: This lecture covers the ON and OFF channels and demonstrates the effects of a glutamate analog called 2-amino-4-phosphonobutyrate (APB) on the responses of neurons in the visual system.
Instructor: Peter H Schiller
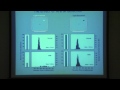
Lec 4: The ON and OFF channels
The following content is provided under a Creative Commons license. Your support will help MIT OpenCourseWare continue to offer high quality educational resources for free. To make a donation or to view additional materials from hundreds of MIT courses, visit MIT OpenCourseWare at ocw.mit.edu.
PROFESSOR: All right, so now today, the topic we are going to discuss will be the ON and OFF channels in the retina. I will provide you with a brief overview again of what we have covered before about this, and then will go on and look at this in some depth. Now before I start on that, let me just very briefly mention again that the syllabus-- which all of you I presume have. If any of you doesn't have a syllabus-- any one of you doesn't have a syllabus, we can provide one for you. What I would like to encourage all of your again is that you do this so-called preparatory reading that appears for each session, which will make it easier for you to follow, and consequently, also memorize the basic facts that we are going to be dealing with.
Alright, so therefore let me get started with the so-called ON and OFF channels. And what I would like to do first of all is to tell you what are the major questions we are going to pose in dealing with this very-- to me at least-- very interesting question of the ON and OFF channels. The first prime question you're going to ask is why did the ON and OFF channels evolve? What is their function? As I've already indicated before, it is rather complicated to have created these two channels, and therefore there must have been a great deal of evolutionary pressures to accomplish this. So that's the prime question we're going to ask.
Now more specifically we're going to ask how the rods and the cones are involved in the creation of the ON and OFF channels. And then you're going to ask how do the ON and OFF channels contribute to the center and surround organization of retinal ganglion cells, because this has been a big issue among many investigators over the past 20 or so years. Then you're going to ask what role do these channels play in giving rise to the transforms that we had discussed the last time in visual cortex. And lastly we're going to ask what are the consequences of blocking the ON channel on neuronal activity and on perception. And this last question then will be successful in answering several of the questions that we pose as to why we have these curious arrangement of ON and OFF channels in the retina.
All right. So now let me first of all talk about the neuronal responses-- sorry-- of the ON and OFF retinal ganglion cells. First of all, if you remember, I told you that back in the 19th century, Keffer Hartline was the first to record from single neurons by dissecting single fires in the optic nerve. And then what he did-- he shone light into the eye. And then subsequently-- especially when I talked the last time about Hubel and Wiesel-- what was done is to use reflected light. And most currently we using reflected light by presenting images on a computer monitor facing the animal or the human we are studying.
Now when Keffer Hartline did this by shining light into the eye, he noticed that there are some says which are called ON cells, some cells that are OFF cells, and some cells which are both ON and OFF. Now his idea at that time was, using that method, that ON cells discharge when a stimulus came on in the visual field, and OFF cells signaled their termination. That was his idea, and that's why I call them ON and OFF cells.
So subsequently, especially when reflected the light was used, a different view had emerged as to what might be the reason for having these kinds of cells. And this was further enhanced by the beautiful findings of Kuffler, when he noticed that there was center-surround organization in these cells at the so-called ON, OFF, and ON/OFF cells responded vigorously when a light was shown into the center, but responded less when the light shown into the surround.
So then people began to do all kinds of experiments to try to study this in further detail, and one of them was to use reflected light, either by using a projector or by using a color monitor. And when they did that-- I'm going to show this to you schematically-- they found that if you had a certain lit background like we have here, you could present the stimulus either by virtue of light increment or by virtue of light decrement. And as I had mentioned to you before, the visual system is dramatically different from the other senses that we have in that we need to be able to see dark stimuli in a background, like letters on a page, as well as light incremental stimuli.
And so this idea then, if it's selected that way schematically, looks like this. An ON cell will fire vigorously when a bright spot appears in the center, and will not fire to a dark spot. If anything, it'll be slightly inhibited. OK? By contrast, when you look at an OFF cell, you get inverse. It fires vigorously. If you would, it gets excited by a dark spot. It gets excited by the detriment in light illumination.
Now if you did the same thing using a much larger spot of light-- that is shown here-- what you see here is that the center-surround antagonism that we talked about fully applies to this kind of stimulation conditions as well. Use a large spot, bright spot, or dark spot and you see that the over responses of the cells-- the responses are much more limited than when you confine the simulation to the center, only further emphasizing this important fact about center-surround antagonism-- this incredible increasing complexity of the organization of neurons that you see already in the retina.
OK, so now we are going to next look at the anatomy of the ON and OFF ganglion cells. And as we look at these various ways of studying the ON and OFF channels, we are gradually going to understand what these two systems are really for. So the first thing is to use this method that I had also already described, namely that you can record intracellularly from a cell, understand its functional responses, and then you can inject a label, and then you can process that anatomically to see what it looks like.
So when this was done, a very interesting new finding had emerged, which is depicted here. Here we have the photoreceptors-- just the tail end of them, if you will-- that connect to the bipolar cells. Two bipolar cells are shown. And then they project into the inner plexiform layer, which we often just refer to as IPL, which is subdivided into two parts-- A and B. And it was shown that the majority of cells arborize in either one or the other of these two sublamina in the inner plexiform layer.
But there were also some cells, which is not shown here, that had arborization in both of these lamina, and that's to anticipate actually the property of those cells, which are called the ON/OFF cells. Now furthermore, it was found-- because these cells were labeled after recording-- that the cells that arborized in subliminal A, or the inner plexiform layer, were so-called OFF cells, and those that arborized in subliminal B, like this one here, and this one here, and this one here, were ON cells. OK? So there was a distinct spatial segregation into the lamina of those retinal ganglion cells that were ON and OFF, and also furthermore, those that were ON/OFF because they had arborization in both of them. So that was the basic finding.
Now when that was discovered, people went on and asked the question, what is the spacial arrangement of these cells on the retinal surface, if you will. And so experiments were done in which cells were labeled, in most cases with the Golgi stain, and then the retina was looked at head on with a microscope. Now the interesting thing about a microscope is, which all of you I'm sure know, is that the higher the magnification that you use, the shorter the focus of the microscope. And in fact, what you can do here is when you look at his head I like that, if you can focus either in sublamina A or sublamina B, and if you do that, since we had gotten these results, you know that whatever focuses into sublamina A would be OFF cells, and whatever comes into focus and the [INAUDIBLE] is coming to focus in B, would be the ON cells.
So then what was done by a fellow called Heinz Wesley is he asked a question, what is the spacial arrangement of these two? Do they respect each other, or what? And so when he did that, here is a good example of what that looks like. Here we have labeled both the ON and OFF cells using the two depth of [INAUDIBLE], and you can see they're kind of helter skelter. Then if he focus deeper, he focus only in the ON cells. It looks like a they're very, very nicely arranged, and that's also true for the OFF cells. And they respect each other. Looking at the dendritic arbors, there's a little overlap among the dendrites themselves of the cells.
So what's this then said is that the ON cells respect each other but don't seem to give a damn about the OFF cells. And the reverse applied to the OFF cells. OK? So as if there were two independent entities. So that was a very interesting finding. And so that was one of the initial cues saying that these ON and OFF cells do different things, and each is a devoted entity to what it does.
All right so now to understand this even better, let's go back and take a brief overview of the retinal connections so we can understand how these systems came about. And the first thing to do is to talk about the photoreceptor basics. And I told you many times-- by now I'm sure all of you know that already-- that all photoreceptors hyperpolarize to light. Then I also told you when photoreceptors depolarize-- that's when they release their neurotransmitter, which is glutamate. And that glutamate acts on both the horizontal cells and the bipolar cells.
And then furthermore, just to look in the details-- this you don't need to remember exactly in these words-- photon absorption by the photopigment results in isomerization of the chromophore from 11-cis to all-trans. To all-trans this causes hyperpolarization, thereby reducing neurotransmitter release. But what you do need to remember is the next step, which says the same thing in much simpler words. It says that the two classes of bipolars, the ON and the OFF, synaptic junction of the OFF bipolars is sign conserving, and that of the ON bipolars is sign inverting. And so as I've told you the last time, as you hyperpolarize and depolarize the photoreceptor, an OFF bipolar cell does the same thing like this, but the ON bipolar does the opposite, OK? So you created a two-ended system-- a double-ended system, if you will-- from a single-ended one that you have in the photoreceptors.
Now, yet another fact that is important to remember is that the ON bipolar cell receptor is mGluR6. That is a specific molecule that exists in most animals, only in the retina, created anew in the course of evolution, indicating what considerable pressure there had to be able to have an ON system. And lastly I should add here also that the OFF bipolar receptors are mGlueR1 and 2 and they follow, pretty much, the way the photoreceptors respond, which means that their activation leads to the opening of channels, causing depolarization. And of course you all know that-- I should restate maybe once more-- that when neurons in the retina that had greater potentials, when they depolarize, neurotransmitters released. When they hyperpolarize, they do not release them. In anything, they stop releasing them.
OK. So now let's look at this wiring again in more graphic detail. Here we have a cone. And the fact is that in central retina, each cone connects with at least two bipolar cells. So in other words, in the retina there are many, many more bipolars than there are photoreceptors, which is amazing if you think about the numbers. I don't know if you remember the numbers. I told you there are more than 50 million cones, and maybe 150 million rods, in the retina. So the retina is an unbelievably complex structure with millions and millions and millions of cells.
All right. So what happens then-- you create these ON and OFF bipolar cells. The OFF ones, by virtue are sign conserving synapse. The OFF, by virtue, are sign conserving synapse. And they connect with two basic classes of ganglion cells-- the ON and the OFF. So the ON and OFF signals, in large part, then are sent separately, especially to the [INAUDIBLE] nucleus. But let's not forget that they also have the so-called ON/OFF cells. And many of them actually end up projecting the colliculus, as we'll talk about later on.
So this is a very basic wiring. They can then add here, as I've already said. This is sign inverting synapse. This is sign-conserving sign conserving synapse. And then we can add the fact that we have horizontal cells that collect signals going sideways on the retinal surface, thereby creating-- hypothetically speaking, at least-- the surround effect, although that we will look at in just a minute in more detail because some people had proposed-- many hypotheses had emerged why we have ON and OFF channels-- and a lot of the hypotheses was to create this famous center-surround antagonism that you see in the retinal ganglion cells. I'll have a model for that in a minute.
OK, so here we have the ON and OFF systems. And again I want to point out to you that they terminate in different sublamina, the inner plexiform layer-- the ON in sublamina B and the OFF in sublamina A, whereas the ON/OFF system looks something like this. It has dendritic arbors. They are ON/OFF ganglion cell in both layers, and connects to both the ON and the OFF like that. So that's how you create these three types of cells at the very simple level.
Now the issue that I just to referred to is how is this surround mechanism created. One hypothesis is that it's created by virtue of the horizontal cells predominantly, as shown in this figure, which is pretty much what you had seen before. So the surround is created by the horizontal cell network. An alternative hypothesis that was for a while popular was that actually the reason we have ON or OFF channels is to create the surround antagonism in retinal ganglion cells by using this kind of wiring arrangement.
I'm not going to go into details about this because to anticipate what I'm going to tell you is that this model does not seem to be correct, and that of course is often the case. We have many, many different models and then one of our tasks is to try to figure out which one is correct. And you know what often happens is that if three models are correct, well there's a fourth one you didn't even think about. So that sometimes happens, and especially when you study the brain.
All right. So now we are going to move on. This is, unless you had read the preparatory material, is something that may puzzle you-- the effects of APB on the responses of neurons in the visual system. So now I'm going to ask you guys as to how many of you actually know what APB is. Oh, I see all these hands raised. Oh my goodness. All right. So nobody knows what the APB is. That's good. So that way you are going to learn something brand new. But if you get a chance and read the preparatory material, then it will be easier for to remember these many, many facts that I'm going to try to impart on you with each lecture.
All right, so APB. APB stands for an artificial molecule called 2-amino-4-phosphonobutyric acid. And to show that to you in some detail, it is shown here-- 2-amino-4-phosphonobuterate . Butyrate-- "ate," as you know, stands for acid. OK? So this molecule, as I mentioned to you before, was invented, if you will, by Watkins and Evans in England, who are molecular biologists. And their game, as I mentioned, is to create new molecules-- and many of which are either analogs or antagonists of neurotransmitters. Now this is a glutamate neurotransmitter that is discharged by many, many cells in the brain, including your photoreceptors. So this is a variant of that.
Now once this molecule was invented, or created, I should say perhaps, people began to ask the question, well what is this variant? What can they do for us? And that was the basic game they all played. You create molecule after molecule after molecule, and then you test them to see whether they can tell you something useful in studying the brain. And it turns out that there's-- I've mentioned before also-- most of those molecules you can throw into the waste paper basket. But the few of them come out and become very, very useful. And this one here-- that's the reason I'm mentioning it to you-- this APB turned out to be a magic bullet.
First of all-- you should remember this-- that this a neurotransmitter analog. So what happens is in a way it acts like glutamate, but it acts really specifically on the neurotransmitter sites of the ON bipolar cells. And what it does-- it fills the receptor site, which then makes those neurons insensitive to subsequent light stimulation. All right? So this then is a remarkable molecule. And consequently, people began to do all sorts of experiments. And it was discovered that when this substance was applied to the retina, indeed shining light into the receptor fields of cells failed to elicit a response. But by contrast, when as part of dark stimulus was present in the receptor field, the cells continued to fire.
So that was a basic finding, and so a method was developed to study this in the monkey. And let me show you what the method is so you can get a sense of what it's like to do experiments like this. So the method was to put two tubes into the eye of an anesthetized, paralyzed animal, through one of which you could infuse a substance, and through the other which the substance could exit.
Because if there were no exiting and you fuse something in here, the eye would swell up, so to speak, and it would kill the cells in the eye. So you got to circulate it. So what you have to do also is to take the vitreous humor and make sure that-- you know that's a jelly-like substance-- to make it so that it was fluid enough to come out of this tube here, as well. So then you could present to the eye either a solution that had no effect on anything, because you made that solution with enough molecular substances similar to what's in the vitreous already. OK?
And then what you could do is you could switch over using this same substance and add a little bit of APB. Now APB is a very powerful substance so you need only small amounts of it to have an effect. And then what you did is you recorded from either the lateral geniculate nucleus, in this case, or from the optic nerve fibers, or from the visual cortex.
And so now you have this magic bullet, and first, however, you want to determine, is it true. What has been found originally in the mutt puppy-- is it also true in the monkey? If you inject this APB into the eye of a monkey, do you really stop the responses of ON-center cells to view light visual stimulation, light incremental stimulation. And the answer is lucky B-- because otherwise I wouldn't be telling you this, of course-- is that the answer is yes.
So here is an example of taking a response. These are cumulative histograms, OK? You've turned the light on, and then turned it off. And you can see here's an ON-center cell responds vigorously under normal conditions. You put APB into the eye, the cell stops responding. And then when the animal recovers from this, you wash it out, so to speak, then the response returns. By contrast, we do same experiment in an OFF-center cell, shown on the right. You see that the cell continues to respond when you put APB into the eye. So this is indeed a magic bullet, which selectively blocks the ON system, and has no significant effect on the OFF system.
So this then opened the gates to study what happens when you do these kinds of experiments-- in this case, in monkeys-- to determine what might be the function of these two systems. And so this then enabled one to test the various hypotheses, which I had mentioned to you briefly before. The first hypothesis you can ask, is it true-- as that model I've shown you the right-- that it is interaction between the ON or OFF systems that gives rise to the center-surround antagonism of cells in the retina. That's one thing you could test.
So to assess that, let me show you what the experimental procedure is. What you do here-- here is a receptive field of a cell. And in this case, this cell-- the recording is taking place in the geniculate-- and this particular cell is one that is color selective. It responds best to a small red spot. And if you hit the surround with a different color, the cell is inhibited. So the way this is then done is you shine the light on the center, then you shine the light on the surround, and then you're in the OFF cycle-- you just hit the surround alone. So this then shown to you here over time. Here's a center stimulation. Turn it on. Then you hit the surround. Then you turn it off. So because-- and the center stays on, and during the OFF cycle, you hit the surround. So this shows the exact manner in which you turn these on and off.
All right. So now the big question is, how do ON- and OFF-center cells respond to this stimulus arrangement. So say you have an ON cell, which would discharge to this. Then the response should decrease because of the surround inhibition. And you'll see the normal response. And the question now-- what's going to happen if you put in the APB? Well there are a number of possibilities. One is that nothing's going to happen. That's unlikely because I already showed you that APB blocks the ON response of the center. The other possibility is that you lose the center-surround antagonism, which would prove that the ON and OFF systems play a significant role in creating center-surround antagonism.
All right. So those are some of the possibilities. And of course a third possibility is that the OFF cells also lose their surround antagonism, although their responses to the center are unaffected. So let's take a look at that. And here are the data under normal conditions. This again is the stimulation arrangement lined up, but you can see here there's a huge response when you hit the center with a red spot, and then when you hit the surround there's a huge inhibition. When you remove that, the response returns. And then during the OFF cycle, there's some spontaneous activity, but the surround activation even stops that. So there's very strong center-surround antagonism.
All right. So now I want you to guess for a minute. What do you think is going to happen when we inject APB into the eye? Think about it for a minute and I'll tell you. I listed some of the alternatives, and you can have a hypothesis of your own of what you think might happen. All right let me show you what really happened, OK? When this happened, actually, the experimenter thought, my god, we lost a cell while we were recording. But then, subsequently when the APB was washed out, you got the same response as before. And so the cell wasn't lost, but the APB was so effective that it stopped the response of the cell completely, both of the center and to the surround.
And now let's ask the question, what happens when you do this, not to an ON-center cell, but an OFF-center cell. Think about it again for a minute and ask what do you think would happen then. OK, so here it is. Here is an OFF-center ganglion cell. And it shows here, using the same stimulus conditions, that in this case, because there's an OFF cell, adding the surround decreases the degree of inhibition turning OFF cycle. And here again the same thing is happening. But the same thing happens under both normal conditions and after APB had been injected. So what this experiment then shows-- and of course one does this with many, many cells-- is to prove that the hypothesis, that the ON and OFF systems play a significant role in center-surround antagonism, is wrong. And because of that, that hypothesis has been eliminated.
All right. So then we can move on and ask the next question. I mean, let me first draw this up again here. All right. Here we have the photoreceptors-- the cone photoreceptors-- the horizontal cells, and the ON and OFF cells. So this alternative hypothesis, that the surround inhibition is due to horizontal cells, therefore, has gained much greater acceptance. OK.
So now we are going to move upstairs and we're going to go to area V1 where we can ask the question, well what were the transforms that we had seen in V1. I presume all of you remember. What are the three major transforms we talked about? Who remembers? Come on. Sure you remember that, don't you-- that we have cells-- most of the cells are orientation specific, right? Most of the cells are direction selective. And most of the cells are selective for spatial frequency. So those are three of the major transforms. The other two we talked about is a convergence of the ON and OFF channels onto single cells in the cortex. And the other was that there was a binocular input to many of the cells. So those were the major transforms we talked about the last time.
And again I want to emphasize the importance of this by telling you that Hubel received the Nobel Prize for those discoveries of how V1 operates. OK? And of course I mentioned before that Keffer Hartline, when he discovered the ON and OFF systems using light that he shown into the eye-- he called them the ON and OFF cells-- he received the Nobel Prize as well. So those are truly, truly major, major discoveries and have triggered hundreds and hundreds of experiments trying to understand better.
And certainly one of the questions that was raised is how does orientation, and direction cell activity, and special frequencies activity, for that matter, arise in area of V1. And one prominent hypotheses was indeed that the reason we have ON and OFF channels is to create these transforms in the visual cortex. So what we can then do, now that one has this magic bullet of the APB, that one can record from a cortical cell. See how it responds when you inject APB in contrast to before you injected it and after injected it. So that's the big question you're going to ask next.
All right. So here is an example of what of cortical cell's response looks like using this kind of histogram when a bar of light, in this case, is moved across the receptive field. Now this is a complex cell. And you can see it gives a vigorous response when the light edge goes across, and then it gives a vigorous response when the dark edge goes across. I'm showing this only for one direction of motion. So that is a natural response.
So now the question comes up, what happens when you inject APB into the eye. A number of possibilities exist. The most basic one I think most of you would buy is that the light edge response is produced predominantly by the ON channel, and the dark edge response by the OFF channel. And if that is the case, then this response should be eliminated by blocking it with APB in the eye. And if you do that, that's exactly what happens. You can see here under normal conditions, you get a vigorous response for both light and dark edges. After APB, only a dark edge response remains. So this then established that these responses to the different edges-- the light edges and dark edges-- are a product of the input from the retina to the geniculate, and then to the cortex from the ON and the OFF channels, which then converge in many cortical cells, as is the case in this particular cortical cell. So that then establishes this very basic fact.
Now the next question we can ask-- what about those transforms you talked about? Let's first of all look at the transform of direction selectivity. So we can take a cell that is, in this case, directionally biased. And you can see what the response is before and after APB is injected. And what you see here-- we move the bar across in one direction, and then we move the bar back across the opposite direction. So the first half shows a downward movement-- the second half, the upward movement. Now what you see here that the cell responds is much more vigorous. It's not 100% direction selective. I showed you some of those the last time. This one is a bias-- about a four to one bias-- much more response to the downward movement than the upward movement. But the cell responds both the light and the dark edge in both directions, and it lines up with the temporal arrangement here, proving indeed that this is a complex cell.
Now then when you inject APB into the eye, look what happens. You eliminate the light edge response here and here, but the cell is still directionally biased. So direction specificity was maintained. And when this was studied in many, many, many cells-- even cells that were 100% direction specific-- blocking the ON channel did not eliminate directionality in the cortex. So that then indicates that the ON and OFF channels did not arise to bring about direction selectivity in the cortex.
And so that brings us to the second transform, which is orientation specificity. So we can do that next. And here we have an example using a very similar procedure. Again, a complex cell when the APB is injected. The light edge response disappears. You move the bar across the different orientations and this is the orientation specificity you get. Now this is calculated here on the basis of only the dark edge response because you want to keep it the same as you're going to do here. Since the light edge response is eliminated, you can see that the cells orientation specificity is also unaffected, meaning that the orientation specificity of the cortical cells is not due to the interaction between the ON and OFF channel.
Similar experiments were also done with spatial frequency selectivity, and again, no effect was found. So this then led to the conclusion that the ON and OFF channels did not arise for the purpose of creating the transforms that we had denoted in our last lecture about single cells in the visual cortex.
All right. So now what we can do is here-- we can come up with a silly model, just to make it memorable, which is to say that the ON and OFF channels flow into the cortex. And these attributes we talked about-- orientation, direction, and spacial frequencies selectivity-- are produced by cortical filters. What you have in the cortex are all kinds of inhibitory interneurons and the interactions among many, many neurons. And that kind of activity that you get there is the one that produces these attributes, not the interaction between the ON and OFF channels that flow into the visual cortex.
All right. So now the next question we can ask, or what happens on APB under photopic viewing conditions. We'll talk about scotopic, as well as photopic, vision. To do this, and to answer how it affects our visual capacities, what we do is to turn to behavioral experiments. In behavioral experiments, what you can do is you can present various kinds of stimuli. You can train a monkey-- in this case, with monkeys-- to see how well they perceive a stimulus, and ask them to make an eye movement to that stimulus.
So when you do this-- testing that hypothesis I've shown you earlier about light increment and light decrement-- what we can do is to train a monkey to first fixate. And then after the monkey fixated, you can present either a light incremental spot or a light decremental spot. The monkey's task is to make a saccade to that target. And of course, those spots will appear randomly in several different locations in each trial, so the monkey doesn't know where he's going to appear. And if he makes a correct saccade to it, he will get a drop of apple juice for a reward.
So that is the procedure. And so now the question then comes up-- how well does the monkey do in being able to detect light increment and light decrement? So if you do that, luckily one gets a very clear cut and dramatic result. Here is the section that I will show you data for light increment, and here is a section showing data for light decrement. So here is an example. What you measure here are two things-- you measure the monkey's percent correct performance, and you measure what the saccadic latencies are to make a saccade to the target. So it shows here that under normal conditions, the monkey's performances is over 90%, and that he has a very nice distribution of saccadic latencies with a mean of about two in 53 milliseconds. Then when you apply APB to the eye, what happens is that the monkey's performance drops dramatically. He is just a little bit above probability, and his latencies are very, very late-- very, very, very long-- 406 milliseconds. So in other words, the monkey can barely perceive a light incremental stimulus by virtue of having blocked the ON channel in the retina by APB.
Now if you do the same experiment with light decrement, what you see here is the monkey's performance remains normal-- about 95% correct. And the latency distribution is also about the same-- two in 49, versus two in 51 milliseconds. So that then says that indeed the ability to detect light incremental stimuli has been devastated by the injection of APB, and therefore raised the idea that the ON and OFF channels are for the purpose of quickly and efficiently detecting light increment, as well as light decrement. So that then was the basic finding that was obtained with this behavioral experiment studying the monkey's performance on being able to process visual stimuli.
Now we are going to get a bit more complicated, because what we talked about so far was under photopic conditions, meaning when your cones were fully functional. So the question is what happens under scotopic conditions, meaning what happens when you're dark adapted. Now we have talked about this before. It was pointed out, initially by Schultze, that we have two basic classes of photoreceptors-- the rods and the cones-- and that the rods are for night vision.
So now the question is, what happens under night vision conditions. And when this was found-- this was truly, truly baffling. And I'm going to show you the data. It showed here under light adapted conditions with the same kind of data-- but shown only as histograms in this case-- the monkey does equally well for light and dark. And when you apply APB, the monkey has difficulty seeing the light incremental stimulus, but has little loss in the light decremental stimulus. OK? But when you do the same thing under dark adapted conditions, when only the rods are operative, a curious thing happens. The monkey doesn't see either light increment or light decrement. In other words, and the way to put it shortly, is to say that the monkey has become night blind. Now there are, among humans, a small population of individuals individuals who are night blind, and what's the situation with them? It's called "night blind" because they just see very poorly at night. And, in fact, when that is known, they only get a special kind of driver's license-- one saying you can drive in the daytime, but you can't drive at night.
So now we have a monkey here with APB injected-- that he's night blind. And also you see the same data reflected in the latencies, just like before. And it shows that the monkey is devastated both for light increment and light decrement by having a tremendous increase-- more than a tripling of latencies for the few trials that he did carry out correctly. So now this being the case, it raises a big question-- and I'm sorry that things are getting so complicated, but the brain is complicated-- as to just what is the nature and arrangement of the rods in the retina, and how does that relate to the arrangement of the cones? OK?
So that brings me to an interesting point. Again, as so often happens, whenever you're confronted with a puzzle, you have all kinds of hypotheses. And then luckily if you do this instead of experiments, you come up with the right kinds of tools. You can eliminate most of them and come up with the right one.
Now, so we can ask, first of all, without talking about APB at all now, simply ask a general question-- what happens to the receptive fields, or retinal ganglion cells, under dark adapted conditions? And what happens is that the receptive fields become larger. Now this initially-- discovered by Barlow in England-- was negated by the other individuals saying, you just got scattering of light-- that's why it's larger. So it was kind of dismissed, but in the end it turned that he was right, as you shall see. The other thing that happened is that the color selectivity response disappears in the retinal ganglion cells. And not only that, but it disappears behaviorally. So when you have a red rose, and you look at a red rose at night when only your rods are functional, it looks like a black rose. The rods cannot process color. OK? They can only process light increment and decrement. OK, again just to reiterate-- the receptive fields become larger.
OK. So the big question is what on earth is happening, what kind of wiring is taking place, or what kind of connections are created to create these two things. Now the second one is the one that's the big puzzle. The first one we can understand because it's been shown that rods are only one type and that they don't carry color information.
Now to study this further, way back when in the 1870s-- 1880s, Cajal did the large number of experiments of this sort. If you remember, I told you that Cajal played a central role in using the Golgi stain, and that he and Golgi received the Nobel Prize in 1906 for their major discoveries about this. So now he argued that the way this happens is that rods and the cones connect differently with the ganglion cells.
And so here is a picture of Cajal. He spent most of his life like this, looking through a microscope, endlessly looking at brain slices, and studying the individual cells-- the shapes and drew them, as you can see his pencil. He is in the middle drawing, actually, a cell there. And so he speculated about this a great deal. And he was just a remarkable person.
And he had written an autobiography. And the way he speaks in this-- translated from Spanish-- but he has an incredibly colorful way of talking-- "had," I guess I should say. And I want to quote some of this, because I think you'll find it interesting and amusing. So he said, "Since this impression received by the rods is different from that taken by the cone, it is necessary from every point of view that each of these specific impressions should be conveyed through the retina by a separate channel." The translation leaves a lot to be desired, but that's OK. So then he went on and said, "When we reason with common sense and lift a war club determined upon vigorous action, nature ultimately hears us." Very picturesque, as if nature had heard anyone, right? "Knowing what I was looking for--" meaning he had a hypothetical bias, right-- "I began to explore eagerly retina of fishes and mammals. Finally as a reward of my faith, there deigned to appear most clearly and brilliantly those two types of bipolar cells demanded by theory and guessed by reason."
What he means by this is that there were two kinds of basic kinds of bipolar cells-- and not the ON and OFF. But in this case, what he was talking about, he was talking about rod bipolars and cone bipolars. He said the rod photoreceptors connect with rod bipolars, and the cone photoreceptors with cone bipolars. So that's what [INAUDIBLE] then established, and that finding was basically correct. But then he went on to say that these two kinds of bipolars hook up with two types of ganglion cells, thereby forming separate channels to the brain. So that was an interesting conclusion, and for many years that was accepted. And interestingly enough, as it so often happens with hypotheses, this conclusion-- even though you had the drawings looking through the microscope showing that there were these separate channels-- turned out to be largely not one-- almost entirely wrong.
Now what do we mean by that? Well people began to do all sorts of very careful intercellular recordings in the retina, and using much more sophisticated anatomical procedures than Cajal was able to use, thanks to the new developments in the field. And they discovered, first of all-- let me back up a second. This is sort of the model that he had proposed. He had the cone model and the rod model, not distinguishing between the ON and OFF. And so the model that then subsequently emerged was that ganglion cells in most of the retina actually receive a convergent input- except in the fovia, because you don't have rods there-- so that you don't have a doubling of ganglion cells-- some for rods and some for cones-- but both the rods and the cones, through separate pathways, feed into the ganglion cell.
So then this was analyzed in much, much more detail. And now I'm going to point this out to you by providing you with an overview of the retinal connections. OK. So here we have the three basic classes of cones. All right? And we have the rods. All right? Then, if you look at the cones, each cone gives rise to at least two bipolar cell-- an ON and OFF bipolar cell. But the rods in most species only have a single kind of bipolar cell, and they can refer to that as an ON bipolar cell, because the synapses are all sign-inverting synapses using the mGluR6 neurotransmitter receptor site. So that's what we have there. Now if you look further down here at the ganglion cells-- and what we have is of course, that we have the ON and OFF bipolar cells from the cones feed into the ON and OFF ganglion cells.
So now the questions are what happens to the ON bipolar cells? How do they connect to these cells since they do not form a separate pathway? And this has been again-- things got very complicated in the course of evolution where it was very important to try to conserve things as much as possible, and that's why we do not have separate pathways for rods and cones. If we did we'd need a huge eye, and we'd need at least twice as many ganglion cells than we have at the present time, which is about a million in each eye.
OK, so what really happened is-- dumb-founding almost-- is that a so-called amacrine cell -- I told you there are different classes of amacrine cells-- one of them is a so-called A2 amacrine cell. That is a cell that receives a direct input from the ON bipolar cells. Now that amacrine cell connects to ganglion cells in two different ways. It makes a gap junction connection with the ON bipolar cells, and it makes a glycinergic synaptic connection with the OFF ganglion cell.
So let's label this. What we have here-- this is inner plexiform layer again, and here is the outer plexiform layer. And what we have here-- just to remind you, that already know-- we have a sign-inverting synapse for the ON, and sign-conserving for the OFF bipolars that connect with the cones. And now we have here-- let's proceed to the A2 amacrine cell that is feeding into these cells from the rods. And what we have here is a glycinergic synapse, so-called. That's a real synapse. It's inhibitory. And then here we have what is called a gap junction.
I'm sure all of you know what these things are. Gap junction is what you call an electrical synapse. So they transform the signal without any neurotransmitters involved. So it directly activates-- in this case, this fiber here-- and drives it. So what you create in the inner retina then here is a double ended system for the rods that is created in the outer retina for the cones. So you create [INAUDIBLE]. Here are the OFF, and here are the ON inputs to the ON and OFF ganglion cells. Now because of this arrangement, what happens is that the size of the receptive fields under dark adaptive conditions is bigger because a bigger range of connections from the rods to the ganglion cells.
So all this is nice wiring that finally, after many, many years of experiments, has been clarified. Sorry it is so complicated. That's just how it is. Explains then the claim that you have bigger receptive fields at night than in the daytime. And now that has been generally accepted.
OK so now the central conclusions that we come to is that ON and OFF channels have emerged in the course of evolution to enable organisms to process light both incrementally and decrementally for being able to see things rapidly and quickly. All right? So that is the prime function. Nature has gone to incredible extent to modify the basic organization of the visual system and the retina to create a way to be able to process both light increment and decrement rapidly.
Now that may provide you with a little cute mnemonic, if you will. Here we have a fish. Fish also, believe it or not, have ON and OFF channels. Now what happens is if below here there is a predator-- a large fish that is trying to catch this fish-- because of the sun shining on it, it reflects the light, and so this fish would see this particular predator by virtue of light increment. Got it? By contrast, if you have osprey up here that's trying to catch this fish, that osprey against the sky would be seen as a dark object. OK? And so now, since this fish has both ON and OFF cells in its retina, it's going to do this. You ready? He's going to escape, OK? So that then, in a nutshell, tells us why we have ON and OFF channels in the visual system-- namely to enable us to process both light increment and light decrement effectively.
And as I've mentioned to you before, whenever you read or write, you see things mostly by virtue of your OFF system because you have dark print or a dark pen on a light background. But of course the obverse is also the case. So this was known for ages and ages. And that's why eventually, instead of having print in which you have a black page with white letters, you now a white page with black letters, because that's much more economical to achieve. And you can read both light incremental and light decremental letters equally well because we have these ON and OFF channels.
OK. So on the basis of this then, we are ready to summarize why we have this remarkable duality in the retina, originating in the ON and OFF channels, whose circuits we by now, I presume, you understand pretty well. So just to reiterate for the umpteenth time, which is easy to remember by now, all photoreceptors hyperpolarize to light. Secondly, the cone driven ON and OFF channels originate at the level of the retinal bipolar cells. OK? For ON bipolars you have a system, which involves sign-inverting synapses. OK? The neuron transmitted by the way of the photoreceptors is glutamate. And you come to the receptor sites-- the mGluR6 receptor is the one that inverts the signal for the ON bipolar cells. And the mGluR1 and 2 is the neurotransmitter receptor site for the OFF bipolar cells.
Now APB-- can important to remember this-- is that glutamate analog. A lot of people make a mistake and think it's an antagonist. It's a glutamate analog. So what happens is that it blocks the ON bipolar. Now let me say just a couple more words about this. We move our eyes about three times a second. Every time you move your eye some mechanisms in the retina wipe the slate clean, so to speak, because if it didn't, then the image that would fall on the retina after you moved your eye would interfere with the image that had fallen on the eye beforehand. So there's an incredibly rapid system that breaks down the molecular arrangement of glutamate and many other transmitters in the retina so that with each movement of the eye, you can see things clearly. Now there is an exception to that, of course, under extreme conditions. We have what is called "after images," like when you look at the sun for a while. Then you will see an after image lingering on in your eye. But under normal illumination conditions, the slate is wiped clean with every shift in the eye. Quite amazing.
All right. Then APB blocks the ON response of retinal ganglion cells. The OFF response and center-surround antagonism are unaffected. APB blocks lighted responses in the cortex, but has no effect on orientation, direction, and spatial frequency selectivity. APB reduces the sensitivity for light increment, and the ON and OFF channels for rods arise in the inner retina. And I should add here that there's only one kind of rod bipolar, which is the ON-type. There are no OFF-type rod biopolars. Then in most primates there are only ON rod bipolars. The rod ON and OFF channels are created in the inner retina by the amacrine cells, predominately by the so-called A2 amacrine cell, as I told you. Lastly, excitatory signals are generated for both light increment and for light decrement by virtue of the ON and OFF channels.
So those are the major conclusions that we need to make about this remarkable achievement of how the visual system works, by virtue of having created the ON and the OFF channels, by virtue of having then done this, by virtue of creating from a single-ended system of the photoreceptors, a double-ended system at the level of the bipolar cells. So that then is-- sorry how complicated it is-- the basic layout of the retina and the ON and OFF channels.
Next time we are going to talk about yet another subdivision in retinal ganglion cells-- the so-called midget and parasol cells. I think I've mentioned to you before that we have several different classes of retinal ganglion cells-- not just ON and OFF. And the so-called midget and parasol cells have ON and OFF subdivisions. And then there are several other cells that do all kinds of other things. We will talk about some of those. But the overwhelming, largest number of cells in the retina are the midget and the parasol, and so they deserve the greatest scrutiny as to why we have those two systems. And that's we are going to discuss the next time.
So that pretty well finishes what I had to cover today, and so if any of you have any questions, I will be happy to try to answer them for you, as long as it pertains, of course, to the ON and OFF channels. Anybody not clear on the rules of how APB works-- that it's an analog, that it fills the receptor sites of the ON bipolar cells, rendering them insensitive to subsequent light stimulation?
Now I should add maybe one more thing here is that unlike the glutamate, which is broken down in milliseconds, this artificial substance-- APB-- there's no natural optic mechanism for it. And so it lingers on, and that's why it is so effective. And it has to be washed out of the eye-- you typically have to wait 10-- 15 minutes for the eye to return to normal because there's no rapid optic mechanism for the APB, in contrast to the glutamate.
Well it sounds like I was reasonably clear. I hope you can absorb this and marvel at the inventiveness, if you will, of evolution to have created this incredible mechanisms that we see in the retina. It's just dumb-founding. Yes, it's just incredible. So that then is what we going to finish with today. And so I will see you Wednesday, and we talk about the midget and parasol systems, which is also very interesting, by the way. And I hope you've enjoyed hearing about what those two systems are for.
Free Downloads
Video
- iTunes U (MP4 - 148MB)
- Internet Archive (MP4 - 148MB)
Subtitle
- English - US (SRT)