Flash and JavaScript are required for this feature.
Download the video from iTunes U or the Internet Archive.
Description: This lecture covers saccadic eye movements in greater detail, including the role of the superior colliculus, brainstem nuclei and cortical structures involved in saccadic eye movement control. Also discussed are the effects of lesions and pharmacological agents on eye movements.
Instructor: Peter H Schiller
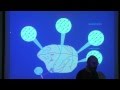
Lec 11: The neural control ...
The following content is provided under a Creative Commons license. Your support will help MIT OpenCourseWare continue to offer high quality educational resources for free. To make a donation or to view additional materials from hundreds of MIT courses, visit MIT OpenCourseWare at ocw.mit.edu
PROFESSOR: All right good afternoon everyone. What I have on the board here is what we're going to cover today and what we had covered the last time. Those in blue here are the ones we have covered the last time and the rest of them, five through nine, are the ones we are going to cover today. Actually the last time I didn't have this last step here, dreaming and rapid eye-movement sleep, if there is time I want to say a few words about that because that's a very interesting topic and brings us to make a few comments about Freudian theory.
All right then so we are going to then start with a description of the cortical structures that are involved in eye-movement control. Now the first thing I want to do is to show you once again the monkey brain. You're already familiar with those items which are on here. Here's V1, here's V4, here's the superior temporal sulcus that contains areas MT and MST and of course here's the central sulcus, principalis, and the arcuate. Another structure involved in eye-movement control is the lateral intraparietal sulcus which is in here, and then another one is the medial intraparietal sulcus but those two perform service similar tasks and so I'm not going to talk about the MIP separately from LIP.
And then here in the frontal lobe we have the frontal eye fields as the name implies of course, it has a lot to do with eye-movement control and then here very close to the midline we have the medial eye fields which also play a role in eye movement control. So now we have all these structures and this is not by any means totally complete, because these deal mostly with saccadic eye movements and to a lesser degree with pursuit eye movements but the fact is it that all these areas that play a significant role in eye-movement control and of course, those people who want to understand eye-movement control need to figure out what these various structures do for you to enable you to look around with the great ease that you can look around with actually.
So anyway let's try a number of views of how to go about finding out the operational characteristics of these areas and furthermore also to find out how they interconnect. Now one approach that has been very useful in delineating the areas in the visual cortex and even in subcortical areas is to use electrical stimulation.
Because it has been found as, as we had discussed like the last time that when you electrically stimulate some structures heavily involved in eye movement control of like the colliculus, at low current levels electrical stimulation can elicit a saccade and by looking at the characteristics of that as we had in the colliculus, you can gain further insight about what the roles are of these various areas.
And of course people have done electrical stimulation all over creation in the visual cortex as well as in other cortical areas, thereby trying to determine whether or not the electrical stimulation elicits a motor response and of course if you do this in motor cortex then you get a motor response and if you do do it in areas which is specifically connected with eye-movement control you get eye movement.
So here is an example of the kinds of things we can do. Here is a monkey brain and of course as we have discussed already, from the brain stem the signal is sent to the eye muscles which provides the so-called rate code and then above that via the superior colliculus and then in the back we have V1 that you're already familiar with V2, LIP, frontal eye fields, and the medial eye fields. So now let's ask the question, what happens when you electrically stimulate these cortical areas? And also to compare that with what happens when you stimulate in the superior colliculus.
So we already know that when you stimulate the colliculus what you get is wherever you put the electrodes in the colliculus and you find out where the receptive field is of the cells that you will be stimulating, that when you then convert and stimulate you get a saccade that brings the fovea into the center of the receptive fields of the neurons that you are stimulating and that is laid out in a nice topographic fashion and that is shown here in a schematic fashion. So that no matter where the eye starts, at any given point, if you electrically stimulate you get the same vector saccade in the superior colliculus. So you have what is called the vector code which if you remember, is quite different from the code that you have in the brain stem where you have a rate code.
So now the question is suppose we now start stimulating these other cortical areas that I had designated in the previous slide and ask the question what happens in those places? All right so if you stimulate the visual cortex in this case V1 for example, you get the same kind of coding operation, you get vector saccades OK, constant vector saccades. Then if you do the same thing in LIP you also get a constant vector saccade and if you do that in the frontal eye fields you still get the same thing. So all of these areas seem to be coding saccadic vectors but now when you stimulate in the medial eye fields you get a very different kind of effect.
Very interesting what you get here is what is called a place code, meaning that when you stimulate in various regions of the medial eye fields, the eye wherever it starts, converges on a particular point which you, we'll call the motor field, OK? And different regions in the medial eye fields have motor fields that generate different locations to which the eye will saccade when you stimulate there. So that's a very different kind of code from all the others.
So now the next question is that you're going to pose is, how do the signals from all these cortical areas get down to the brain stem? So what kind of experiment do you think you would want to do to get some easy answers to that? So to, to perhaps highlight that some people thought, that the signals from all of these areas go down to the colliculus and then the colliculus sends its signals down to the brain stem. So if that's the case, what would you, what experiment would you do?
Well the experiment you would do is you would remove the superior colliculus OK? And then again stimulate all of these areas. If that hypothesis, that all these areas send their signals through the superior colliculus of the brain stem is correct, then you would no longer get any saccades when you electrically stimulate in the cortex at any of these sites. Got it? All right now, let's see if an experiment like that had been done and yes, yes it has been.
So here it is, here we're going to remove the superior colliculus. All right think about it for a minute, think what you would hypothesize will happen? Well what happens is quite dramatic. When you stimulate in V1, V2, and LIP you no longer get a saccade. Somehow the signals to generate the saccade from these areas seem to be going through the colliculus because once the colliculus is not there those signals are ineffective.
Now what happens in the frontal lobe? All right what happens there is quite interesting. You still get saccades and you still get the same coding operation. You get a constant vector code when you stimulate the frontal eye fields and you get your place code in the medial eye fields and you get that at the same old threshold. So that discovery then resulted in the hypothesis first of all that these posterior areas send the signals to the brain stem from the colliculus which are called the posterior system and the ones in the anterior portions of the brain from medial and frontal eye fields seem to be able to gain direct access bypassing the colliculus to the brain stem is because they're still effective when you stimulate there and so we can call that the anterior system.
All right, now of course these two systems need to talk to each other, which they do, there are plenty of connections there because of course, if you will, the left hand, it's got to know what the right hand is doing.
So anyway this is then a very summary arrangement and now we can proceed to ask some questions about just what do these various areas do? And to understand that data we need to look into more detail about the nature of electrical stimulation and compare that with the nature of eye movements made to visual target. So let's look at that next time.
So we can look at the effects of paired electrical and visual stimulation. All right so, the first thing we're going to look at is what happens when you stimulate two sites say in the colliculus at the same time? And if you remember, medial is up and lateral is down. So if you stimulate each of those alone you get here in number one you get an upward saccade, you simulate number two you get a downward saccade.
Now the question comes up, what happens when you stimulate both at the same time? Well the number of hypotheses that have been proposed and by now it's been well established that what happened is that you get a vector average saccade not vector summation, but vector averaging OK?
Now, to prove that that is indeed vector averaging if you take the same experiment but you put one electrode in the anterior portion and the other electrode in the posterior one of the colliculus, this one of course generates a small saccade and this one a large saccade. So if its vector averages, you should get an in-between saccade. If your vector sums then it should get a bigger saccade than either of those and you indeed get a vector average saccade.
So depending on this arrangement, or this arrangement, you get the same vector average saccade because the only thing that's happening here is you excite the neurons in the colliculus by virtue of this electrical stimulation. Now think about that for a minute. If this were the case with visual stimuli, suppose two visual stimuli come up, it would be a total disaster if you made an eye movement between the two of them wouldn't it?
So somehow there's some mechanisms in the brain that force you, force you, I guess that may not be the right word, achieve the ability to select one or the other of the visual targets accurately and not be vector averaging it. Now to accomplish that logically speaking, what you need is some inhibitory circuits and the mechanism for selection and then a decision as to where to look.
All right so to highlight that let me just say one more thing about electrical stimulation. This is true what I told you about vector averaging with electrical stimulation even when you stimulate the two, the two superior colliculi or two different locations in the brain each of those gives you an eye movement into the left or right hemifield and yet they also vector average.
So now let's look at what happens when a similar situation is used in a real experiment where the monkey looks at visual targets and so what you do here is you present these two targets at the same time. And so the monkey in a sense has to make a decision or you have to make a decision as to where to look.
And as long as there's a nice big separation here which is 90 degrees in this case, what you can see here are the real eye movements that half the time the monkey looks to the left, half the time to the right and it makes very very few in-between saccades. Whereas if you electrically stimulated at these sites in the colliculus you'd get a vector average saccade just like what I've shown you here OK?
All right so now that being the case, we can move on and ask some additional questions about what happens when you bring the two stimuli closer together, all right? The closer together they get the more difficult it is to make an independent decision as to whether to look to the left or the right target that comes up above the fixation spot.
So here's an example of that, actually what I'm going to do is this. Let me delay for a little bit what happens with that and first I want to tell you about what happens with various kinds of lesions that you make on eye movements and then we'll talk about this question of the angular separation between the two visual targets. So therefore let's do-- the reason I want to do this first is to give you sort of a real sense when you do very informal kind of testing and by informal testing what I mean is that you're going to see a monkey actually perform eye movements OK?
So here we are, here's a monkey, here's his brain so to speak and this is what we call of course an intact animal and now what I'm going to do, I'm going to start the movie for you to see what kinds of eye movements he makes when we present some apple pieces for the monkey to eat. OK are you ready? Here it is.
Can everybody see this OK?
So you can see that when an apple piece appears the monkey makes a saccade to it and grabs it and stuffs in his mouth and eats it. OK so that's what a normal monkey does with his fully intact, functional brain.
So now we're going to ask the question, what happens if you take out the colliculus on one side, meaning a unilaterally lesion, what happens to the eye movements the monkey makes subsequent to such a lesion? Are you ready for that? Here we are.
It's the same monkey. You can see that his eye movements may be a little bit slower perhaps but he still looks to the left and to the right quite well. He tends to sort of look towards the side of the lesion when there's no stimulus OK, but other than that he seems to make really rather, rather good eye movement in spite of the fact that he has a colliculus missing on one side.
So what that means is that just looking at what the monkey does in this qualitative manner is not going to tell you too much about what these various structures do and so consequently you have to carry out some more refined experiments to determine what kinds of deficits do arise when you take out the colliculus, you take out other cortical structures for the generation of eye movement.
So let me first of all tell you about a really interesting finding that was made that had nothing to do with the colliculus at the time it was made, was a strictly behavioral study much of it done on humans. What was done is first of all a fixation spot came on and then a single target appeared and often what was done is that the fixation spot was turned off just a few milliseconds before the target came on and on each trial it appeared someplace else OK?
So then you've collected a lot of data to see what is the nature of the monkey's eye movements and in particular that initial study examined by Fischer and Boch, examined what the latency distribution was of the eye movements made and they made an incredible discovery that they subsequently generated hundreds of studies published in numerous journals, and this is the discovery.
Here we have the latency of saccades made and here's a number of saccades and what you get is a bimodal distribution of saccadic latencies, amazing. They call the first mode, which takes place in the latency of average latency for bright stimulus over about 100 milliseconds, called express saccades. The second mode they called regular saccades and that took about I don't know, 135, 140 milliseconds on the average.
So that got this bimodal distribution and so people said, my goodness what could this be due to? How do we explain this effect? What other cortical or subcortical mechanisms that give rise to a bimodal distribution of saccadic latencies? As I've said a huge number of experiments had been carried out to find this out. So when one group of investigators saw this phenomenon and they did experiments with monkey's and they found this is a real effect everywhere, these findings were initially made in Germany and even, even in the United States you get a bimodal distribution of saccades in both humans and monkeys.
So once it was established as a really solid effect people began to speculate as to what are the neural, underlying mechanisms? And as I've told you earlier it was noted that based on those lesion studies of the colliculus which eliminated saccades from the posterior portion of the cortex but not from the interior, that there is a reasonable hypothesis involved in proposing that you have a posterior system and an anterior system. And so when people saw this they said aha, now we know why we have these two systems. One is for making rapid saccades and the other is to making regular saccades. And so they proposed that the posterior system does one of these and the anterior system does the other.
Well that was a nice hypothesis but so often when it comes to hypotheses as to how the brain works, often most of the time the hypotheses end up being wrong and so what you need to do rather than just hypothesize is to actually carry out experiments to test the hypothesis. So what is the test of this hypothesis? What would you do as an experimentalist? Well, yes.
AUDIENCE: You could ablate either posterior or the anterior pathways.
PROFESSOR: Very good you could ablate. All right so one thing you can do first of all you can ablate the superior colliculus even though when you saw that just with regular easy test just filming it, you didn't see much of a deficit. You sort of sense that maybe the monkey's a bit slower but other than that it wasn't that clear. But if you take out the colliculus then you eliminate the posterior system in essence all right, and so the question is what happens when you do that? And then you can ask the question what happens when you take out some other cortical areas? So let's look at this.
Here we have a monkey 10 weeks after the colliculus has been ablated, we're talking about a major effect here that doesn't recover, and you took out the colliculus on one side on the left OK, so that controls rightward saccades and therefore when you look at the leftward saccades that's to the intact side of the brain you get the usual bimodal distribution of saccadic latencies. But then when you look at rightward saccades Lo and behold, you don't see a single express saccade and even the regular saccades have a longer latency than the ones to they intact side and these are collected at the same time.
The monkey's sitting there with his head fixed and sometimes a target appears on the left sometimes on the right and you collect hundreds of thousands of trials that way and you test this over various time periods and this is at least 10 weeks, 2 and 1/2 months after the lesion and even if you test the monkey a year later you still get the same effect. So this clearly points out the fact that your ability to make these rapid reflex-like saccades is something that's got to go through the superior colliculus OK?
So now we can ask the question, well, what about if one makes lesions in the frontal and medial eye fields? All right so think about that for a minute what would you predict? All right so here we go. We take out the frontal eye fields in this case, ready? Oops let me go back. We take out the frontal eye fields but to give you a sense of this overall effect first of all let's just do the same informal testing that I've shown you before with the colliculus and see what the movie looks like OK?
Here's a monkey not as handsome as the other one, but what you do here is a person behind there sometimes just presents a target and sometimes just moves it around so the monkey tracks it, can you see that? And what I shouldn't have told you which one I feel have been ablated.
Look at how nicely he tracks on both sides, grabs it, puts it in his, stuffs it in his mouth and so the monkey seems to be perfectly fine with making saccades to either side and perfectly fine making pursuit eye movements either direction, indicating that this informal testing doesn't reveal anything truly obvious about the deficit that you have in eye movement control when you take out the frontal eye fields. So therefore you again need to go on to carry out some more careful experiments to obtain some detailed quantitative data.
So let's look at that. First of all let's go back and look at express saccades. You take out the frontal eye fields and Lo and behold, you still get express saccades indicating that that is definitely specific for the posterior system even further if you do this experiment what you find if you take out both the medial and frontal eye fields you still get it, you still get your express saccades.
So clearly these two areas are not directly involved in generating quick rapid saccadic eye movements and it's still bimodal so that initial hypothesis that the first mode is the colliculus and the second posterior system the colliculus and the second mode is the anterior system is clearly totally wrong.
All right so that's what then happens and once this has been done, you say, well, you've got to find something that the frontal eye and the medial eye fields are doing. So let's come up with some other experiments to see whether the monkey is selective for anything else when it comes to lesions of the frontal and the medial eye fields.
All right so one thing that had been proposed is that maybe the important factor is some high level eye movement activity such as making saccades in quick succession to successive targets that are out there OK? So the way that can be done quantitatively is you have the monkey first fixate OK, and after they fixate you present the target and then you present the second target. So the monkey has to make two successive saccades and what you can do is you can vary the temporal delay between their succession.
So the monkey then has to make a plan to makes two saccades because these two stimuli appear before the monkey starts his initial saccade when the temporal interval is short. So then the monkey somehow knows it's easier to do, he has to do this even though he starts a saccade only after the two targets have come on when they are presented indeed in the short, the short latency.
So that's what happens and of course in different trials you have different kinds of pairings like that and if you do that what you find is very interesting here are the four conditions, this shows the monkey's performance 18 weeks post left frontal eye field lesion and this shows it 60 weeks after and it shows this to the intact side and the side where the frontal eye fields are missing and what you can see very dramatically is that the monkey really has difficulties in making a plan to execute two saccades in a row. Quite dramatic effect significant [INAUDIBLE] one level, of course indicating that the frontal eye fields play a role in planning sequences of eye movements.
Now then another, well this I want to show you, what happens with the, when you compare the effects of the frontal eye field lesions and the medial eye field lesion and this is done over various sequence durations and for several weeks and what you find is that in both cases there is a recovery but the effect is much, much more dramatic with the frontal eye field lesion than a medial eye field lesion. So indeed there's for the frontal eye fields we can say the frontal eye fields play an important role in planning sequences of eye movements which is sort of a high level activity in executing saccadic eye movements.
Now then what we can do is to examine what about making a decision of where to look when more than one target comes up and the simplest form of that is that you present two targets like that OK? And so the monkey has to make a decision am I going to look to the left or to the right? And then what you do is you can vary the temporal delay between the two like that, or like that OK? And then you can do that again either to intact parts of the visual field or those where either frontal eye fields or some other structure is missing.
All right so let's look at what happens. Here is what we have is the intact monkey this part I've shown you before. All right this is when the two targets come on simultaneously and in this case the left target comes on 34 milliseconds before the right and here's the reverse and what you can see that even a 34 millisecond delay causes the monkey to very much prefer to go to the, to the target, make a saccade to the target that had appeared first. So that's what happens in a normal intact monkey.
Now we can ask the next related questions still in the normal intact monkey, what happens when you put the two stimuli closer together? OK so here's an example they're, now they're separated only by 40 degrees and here the data again it shows that when it's, in this case 67 milliseconds apart the monkey chooses almost exclusively the target that comes on first.
When they're simultaneous what happens is interesting, you get some so-called vector average saccades that you always get with electrical stimulation. So those you get, it's still a minority of the saccades. And the closer you bring the two together, the more frequent will be the vector average saccades. When they're only separated by set 10 degrees they will be all vector average saccades So this is what happens in the normal monkey you get this nice bimodal distribution and vector average saccades when the two are simultaneous.
Now let's ask the question what happens when you take out a cortical structure in this case the frontal eye fields and here we are you take out the left frontal eye fields. These above are the same the data I just shown you adding a bigger delay here, just to make it even clearer that by that time the monkey never chooses the target that comes on second.
And here we have the monkey after left frontal eye field lesion and look at what, what happens. What happens is that the monkey at 0 chooses 100% saccade to the intact side OK. There seem to be a little bit of a shift here and then when they're 100 milliseconds, then you have an equal distribution.
So to equalize the choice that the brain makes you have to now because of the missing frontal eye fields, present one of the targets a 100 milliseconds earlier on the affected side to get the same kind of distribution that you get in the intact monkey. So this further highlights the fact that the frontal eye fields play a significant role in making decisions about the selection of visual targets.
All right now we can look at this more quantitatively. This shows the distribution of choices OK, to the left target, this is the intact monkey preoperative and then when you take out the left frontal eye fields it's a huge movement over the equal choices has to be about 130 milliseconds separated with the affected side getting the target a 130 milliseconds earlier. Then if you keep doing this there's some recovery but even four years later you still have a huge effect.
Now you can ask the question what if you do the same experiment in the medial eye fields? And so if you do that you first of all get a small effect to begin with and after just 16 weeks there's full recovery. So obviously the medial eye fields don't, do not seem to play a central role in making decisions as to which target to look at when more than one target appears in the visual field.
All right so now having done that quantitative work indicating that the frontal eye fields play an important role in target selection and the sequencing of eye movements. We can move on and ask the question, well we talked about the posterior system and the anterior system. Is it true that there are these two systems? Or are there many more systems that we're not aware of?
This should remind you of the fact that it had been proposed when we talked about extrastriate cortex that area of V4 essential for processing high level activity including color, whereas the area MT and MST play an important role in emotion and so it was purported that these two major systems in the posterior cortex, the medial and lateral if you will.
So the question was raised if you remove both of these areas, meaning the gateway to these areas, V4 and MT what happens? And when you do that they still the monkey's able to do a lot of things indicating that we have more pathways from V1 than just these two the anterior, the medial and the lateral.
So now the question is what about when it comes to this eye movement control? We should do the same kind of experiment. We should remove the colliculus that supposedly eliminates the posterior system and then we should remove the frontal eye fields bilaterally, that eliminates the anterior system. So now the question is what happens when you do that? And again, we going to turn to the informal test, meaning we going to, we going to take a movie of the monkey who has these areas removed. Think about it for a minute what do you think's going to happen? OK so here's the monkey. Ready?
The monkey sees well, he makes his movements with his hands quite accurately but what, what happens to the eyes, you watching the eyes right? No eye movement. Everybody see this? Should I show it again?
The monkey makes no eye movements. He cannot make eye movements because his natural tendency would be just like I'd seen in the previous movies that he makes eye movements to the apple pieces so he can grab them. He still pays attention to them everything is fine except he doesn't move his eyes because he can't move his eyes as a result of having eliminated these two systems and because of that we can say with confidence that it's, as far as the visual as far as the ocular motor system is concerned we indeed have these two major pathways which when they're eliminated eliminates their ability to move, make saccadic eye movements.
OK now let's go back to the effect of electrical stimulation to gain further insight about what these various areas do and what you can do in these experiments is not only to stimulate at a high level to elicit a saccade but you can simulate at a lower level and then pair that with the appearance of a visual target. This then enables you to see whether there's summation here or if there's interference, so let's look at that.
All right here is again a monkey brain and what I'm going to tell you is what happens when you do this experiment in V1, in LIP, and in the frontal eye fields, even maybe I think I may have something also in the medial eye fields.
All right so let me describe the experimental procedure for you so that you understand how these kinds of experiments are conducted. You put an electrode in to any of these structures and you find the receptive field first all right? Once you've found the receptive field you electrically stimulate then you confirm the fact that the electrical stimulation brings the fovea into the receptive field of the simulated neuron's when you do this in all the areas except in the medial eye fields.
Then what you do is you actually present the visual target there OK? And lastly you present two visual targets and you electrically stimulate to see how it biases your choice as a result of this in most cases sub-threshold electrical stimulation.
So if you do that what you find is, first of all, if you do this experiment with a intact monkey without stimulation you find say a receptive field here and then you simply see what the monkey's choices are left to the right and what you plot here is the saccades made to the target in the receptive field and what you can see is what I've shown you before namely when the targets are simultaneous then the monkey chooses left or right with equal probability.
Now we're going to add the electrical stimulation and ask the question can we shift the curve to the left? If you shift it to the left, that means that the electrical stimulation facilitated the choice and if you shift to the right it means that it caused interference, it lessened the chances of the monkey making a saccade into that area, meaning the stimulation created inhibition. Got it?
All right, so here's an example in the lower layers of V1 remember in layer six of area V1 is where you have your complex cells that project down to the superior colliculus. So here if you stimulate at sub-threshold levels and some of these are very low levels only 7 1/2 and 10 microamps. OK, really very, very fine currents using themselves going to elicit an eye movement. So if you do that the stimulation created a significant, highly significant facilitatory affect. This is in the lower layers. Now if you do the same experiment in the upper layers you get the opposite effect we get a gigantic even-- look at this five and 10 microamps, --even at that low, incredibly low level you get a gigantic interference effect.
So that says that there's a complex interplay in V1 in the decision process that arises as to whether you're going to look at a target, or whether you're not going to look at a target. Now we can do the same experiment in LIP and in some regions you get this huge facilitatory effect and in other regions you get an inhibitory effect, so that's LIP. So therefore this structure also plays a significant role in deciding whether to look at or not to look at a visual stimulus and here it shows that in LIP as you increase the current you get a gigantic increase in the latency in these inhibitory areas with which a saccade can be generated indicating that LIP plays an important role in whether you're going to look at a target or whether you're not going to look at target.
OK and then if you do the frontal eye fields everywhere in the frontal eye fields you get a huge facilitatory effect and in the medial eye fields you also get a facilitatory effect as long as the motor field is where the visual target appears. But now you can remember what I told you about the medial eye fields the fact is that they have a place code. So one can do a different experiment in which instead of presenting the target in the motor field you can present the fixation spot there.
So here again to remind you, we have this place code. So now we do this experiment, just like what I've shown you before just to repeat it, in this case the target appears and that causes a facilitation in this case we put the fixation spot in there and the location of the target's just displaced and when you do that you get a huge inhibitory effect because somehow the electrical stimulation forces the animal keep the eye at the location where the motor field is in the medial eye fields and this is a very important point and it means that the medial eye fields plays a significant role in deciding how long to look at a target before making the next saccade.
OK so now we going to, there's a lot of facts, so and you're going to get some more lot of facts. So now I'm going to summarize what I told you about the effects of sub-threshold electrical stimulation OK? If you stimulate in the upper layers of V1 and V2, V2 I should add to this you get interference, in the lower layers you get facilitation in V4 there was no effect I didn't talk about that before in LIP you can get both facilitation interference and also fixation increase. In the frontal eye fields you get facilitation and the medial eye fields depending on how you set it up you can get facilitation if the target appears in the motor field and you get inhibition when it appear, when the fixation spot appears in it. So that's the basic summary of these effects.
Now what these findings indicate that somehow inhibitory circuits are essential in our ability to make saccades to selected visual targets and therefore what we want to do is to examine what happens when you use various kinds of pharmacological agents that either facilitate or increase inhibition. So to explain that then let me first of all point out to you that if you take again the whole brain and you look at the colliculus and you look at V1, you look at LIP, you look at the frontal eye fields you can study these areas by injecting two kinds of pharmacological agents, bicuculline and muscimol some people believe you've heard of this as muscimol I call it muscimol.
At any rate you all know I'm sure but this is bicuculline is a GABA antagonist meaning that if you inject it OK, it stops the effectiveness of inhibition by GABA. Muscimol on the other hand is a GABA agonist meaning that if you inject it you increase inhibition all right? Now I'm sure that all of you must have had enough of a background in biochemistry to know what these two agents are.
So now what we can do is we can inject either one or the other of these agents and assess two things. First off we assess eye movements and secondly we also have to obviously assess how it affects your visual ability. So let's move on and do this.
The first set of experiments done with this was by Hikosaka and Woods many years ago in the colliculus. So what they did, very clever beautiful experiment, is that they would put a microelectrode into the colliculus and then initially just stimulate to see what kind of eye movement you get and then they would inject either muscimol or what's the other agent?
AUDIENCE: [INAUDIBLE]
PROFESSOR: Very good and see what happens. Now which of those causes more inhibition?
AUDIENCE: [INAUDIBLE]
PROFESSOR: OK, so let's look at that then. If you electrically stimulate just like I've shown you before you get a constant vector saccade no matter where the eye starts you get the same vector. Now the question is what happens to the spontaneous eye movements of the monkey when you first inject muscimol OK? Which is an agent that mimics if you will your GABA, meaning it increases inhibition and what happens is that the monkey hardly ever makes a saccade with the vectors represented by the area that has been injected.
By contrast if you inject bicuculline the monkey keeps making saccades with that vector even when there's nothing out there like the release of area for inhibition. And the signal is sent down to the brain step move your eye, move your eye, move your eye, move your eye, that's what happens. So to show this in more detail then we can ask what happens when you use two behavioral tasks OK, the so-called paired target task that we already talked about quite a bit and you talk about a visual discrimination task and that one is that you're already familiar with the so-called audited task you present several stimuli one of which is different from the others.
All right so here is an example of the paired target task. What we do here again we vary the temporal asynchrony between the two targets just like what I've shown with the electrical stimulation and this is the monkey's normal behavior when the two targets are simultaneous the monkey chooses each randomly.
Now let's ask the question what happens is again to remind you, if it goes this way the curve goes this way it's facilitation if the current curve goes that way we get interference. So let's ask what happens first of all, no let me also tell you about the oddity task just to make sure that you have it. All right that's the oddity task you know that already.
All right so we can now go on and first examine what happens with muscimol or muscimol or whatever you prefer to use OK? Well again what, what does this agent do? Does it do excitation or inhibition?
AUDIENCE: Inhibition.
PROFESSOR: Very good, inhibition because it mimics GABA all right? So let's look at what happens. Here we have a normal monkey same experiment as before, here is the this is V1, here's a receptive field you present one visual target there, the other there, then you vary the temporary asynchrony between them, this is what happens in the normal case OK?
Now you're going to inject the muscimol and ask yourself the question, we increased inhibition what do you think is going to happen? Well it should be pretty obvious, what happens is you get gigantic inhibition the monkey practically never looks at here because that area is not being activated by the visual stimulus because of the inhibition. Then if you do this over time, even four hours later there's a huge, huge effect but by the next day the monkey recovers luckily so one can do this experiment several times because this agent is something that washes out of the brain.
All right so now let's look at what happens in the frontal eye fields. Same experiment, all right, just a different location with the electrode. And what you get, this is your preinjection. And once again, after the injection, you get a big interference effect which recovers by the next day. So that is what you get that with that.
Then let's examine what happens in LIP. Curiously in LIP there was no effect.
All right. So now let's next turn to the so-called oddity task meaning several stimuli, one of which is different from the others and what is the monkey's ability to choose the different stimulus. So if you do this with a muscimol injection there's a huge deficit for V1 that you would expect because it destroyed the monkey's ability to analyze the visual stimulus that appeared in the receptive field. Then if you do the same thing in the frontal eye fields you find a mild deficit and then if you do the same thing in LIP you get no deficit at all.
So this is what then happens with the oddities task and now what you can do is ask what happens when instead of muscimol we're going to inject bicuculline? And we're going to go through the same procedure as what I just shown you and so we start here in V1 and when you do that think about it for a minute, now you're facilitating supposedly because you're eliminating inhibition what do you think you would get?
Well, you'll be in for a surprise, what you get is gigantic interference again because putting bicuculline in also screwed up you're ability in the visual cortex to analyze the visual percept. This again recovers over time by the next day it's back to normal. Then if you do the same experiment in the frontal eye fields what do you think's going to happen? Can you predict what's going to happen just looking at this slide? Why do you think this is such a big empty space here huh? OK well look at that, when you put in bicuculline we get this incredible facilitation.
OK the monkey just like in the colliculus barely can help himself to make a saccade into the field that has been disinhibited OK?, and that again recovers, bicuculline is washed out more rapidly than muscimol and by the next day certainly is back to normal. Now we can do the same thing just looking at the eye movements themselves to further highlight what I've shown you before.
If you'd put bicuculline in OK, in the frontal eye fields the monkey cannot help but make saccades with similar vectors that are represented by the neurons in the injected site and that's why you have all this is its full stack of saccades buh, buh, buh, buh, bang the monkey just can't help but makes saccades because the signal to make the saccade has been disinhibited.
All right so then you can ask what happens in LIP and when you do that you get no effect at all, and if you do now the bicuculline injection with the oddities task we can once again ask well what happens with that? And then once again as I've already indicated, both bicuculline and muscimol cause a major interference in your ability to select the odd target, in other words to visually discriminate, but if you do the same thing in the frontal eye fields and in the medial and LIP you get no effect at all.
So what this then says to summarize all right is the following. We talk about target selection, which was a two target task, individual discrimination which was the oddities task and here we have muscimol and here bicuculline. So if you do that in the frontal eye field, in V1 first, you get interference, you do it in the frontal eye fields you get interference of muscimol with great facilitation, with bicuculline and LIP has no effec.
And then, just to remind you, it has already been shown by Hikosaka and Woods, that you get interference of facilitation in the superior colliculus with these two agents. You do the same thing with visual discrimination, you get a major deficit in V1 for both and you get a mild effect for both actually no effect really with bicuculline in the frontal eye fields and no effect at all in LIP.
So these manipulations then gives you sort of a sense of what these various areas do in the generation of eye movements that involve not only just to make a saccade to a target but to select targets individual field, make a decision as to where to look, and also to decide when to look, because any time you make an eye movement and I should've mentioned that more thoroughly before, you look at something and how long you look at it depends on how long it takes you to analyze what you're looking at. Now in most cases it takes you maybe I don't know 200 milliseconds or less to say, oh yeah that's letter a or whatever and then you say it's your brain mechanisms tell you, OK, now you know what it is. It's OK for you to move your eye.
OK so that's involved and then thirdly, you have an important task in making sequences of eye movements so that when you look at a picture like the movie I showed you in the beginning in the previous lecture, when you look at something then you make a decision as to where to look next, where to look next and if you keep doing this for I don't know 20, 30 saccades then you get to a state where you say oh now I understand the picture as a whole.
All right so now we're going to summarize what the tasks are in a very simple situation and what brain structures are involved in it. First of all let's imagine that you're looking at fixation spot designated by a. Let's assume the two stimuli come on and that means that you have to make a decision as to what those two targets are, you have to identify them because you have to select one of those OK? Of course in most cases you're talking about many targets that are out there this is a highly simplified version.
The next step is to decide which of these two targets now that I know one says a and the other says b, which of those two targets, actually b and c in the picture here which of these targets should I look at, and so you make a decision all right, that generates excitation and inhibitin, inhibition through excitatory and inhibitory circuits, and then you decide OK we going to look here and that means that you have to decide which one not to look at in addition to deciding which one to look at, because you've got to make an accurate saccade so you don't want a vector average.
All right. And then what you need of course is a map, if you will, of the motor field so that you can generate the appropriate direction of the saccade so that you can decide where you're going to look at and then lastly as I've mentioned already before, you also have to make a decision as to when to make that eye movement.
So now in a very summary fashion we can talk about the various brain areas involved. Quite a number of different areas are involved in the decision as to what these two stimuli are, they of course involve much of the visual system including also LIP and several other areas. Then, then you have to make a decision as to which one to look at, again several areas involve notably among them are the frontal eye fields, LIP, and also the medial eye fields.
Then you also have to decide which ones not to look at, that it was largely the same areas and then you need of course a topographic arrangement to know where things are and that you can find in many areas, including V1, V2, the frontal eye fields, and the colliculi which are laid out in a nice topographic fashion, and then lastly LIP is important for that, and I think to some degree also the medial eye fields but I'm not sure about that to decide when you should generate saccadic eye movements.
Well that's very nice and makes you realize that even though we never think about making eye movement all this stuff is going on three times a second it's amazing. So now you're going to look at what the various visual areas and ocular motor areas are that play a role in this OK, and so we're going to create a summary diagram. So here we have, that's the first one I shown you, which has a rate code that from the brain stem connects with the eye muscles and activates them.
Then we have the superior colliculus, now the superior colliculus is under strong inhibitory control all right, and they're several inhibitory circuits that are involved in that and those include the substantia nigra that sends inhibitory circuits down to the colliculus that then prevents the colliculus from generating an eye movement because it is under inhibition, because every time you look around thousands and thousands of impressions impinge on the colliculus and you only want one of those to actually get down to the deep place, the colliculus, to generate an eye movement.
Now then the substantia nigra is under the control of several neural structures which, many of which go through this so-called basal ganglia. Now we can expand on this and look at the visual input to do this. We already talked about this a lot you, pointed out to you that you have these three major, many, many different types of ganglion cells. The three major ones we talked about are the midget parasol and the so-called W-cells that go to the cortex. And the W-cells also project directly to the colliculus.
Then from layer five in the visual cortex you have the cells that project to the superior colliculus and the intermediate layers and that down flow is controlled predominantly by the parasol system as we had described. Then we have all these other areas that V1 projects to, V2, MT, V4, and so on some of them dominated by input from the parasol system and others get input from both, and those in turn project to the parietal and temporal lobes and those in turn have an important influence on the inhibitory circuits through the basal ganglia and the substantia nigra.
Now let me finally come to the frontal lobe, the frontal eye fields, and the medial eye fields and they in turn have direct access to the brain stem and also connect to the superior colliculus. Now this is still not the whole story because what you have in addition is a bunch of interconnections among numerous cortical areas they talk back and forth to each other that enables you to make these decisions as to where to look next.
Now if you think this is complete you're still wrong because now what you have to realize is that all this circuitry is also one that receives input from several other areas, with the auditory system that you're going to hear a lot about later on in the course, the somatosensory system, the olfactory system, the smooth pursuit system that we'll mention a little bit next time, the vestibular system, the accessory optic system we'll talk about next time, and the vergence system.
So all these fit into this incredibly complex circuitry already and essential elements in your ability to move your eyes about. So I think you need to realize therefore that something even as simple as just moving your eyes about it's an incredibly complicated system involving many structures and involving excitatory and inhibitory circuits, interconnections it's just, it's almost dumbfounding.
So that then is the essence of these connections, and what I want turn to next I think we still have a little time. Before I summarize our results for today, I want to say something about dreaming and rapid eye movements.
I think all of you know that every night you sleep you dream and what you also know that has been discovered more recently is when you dream you make rapid eye movement so it's called REM sleep, and so the question arose why do we dream? Why do we have REM sleep?
Now, the major influence as to why we dream comes from the work of Sigmund Freud who published a famous book one of his really great works called, The Interpretation Of Dreams, which was the original version in German was published in 1900. OK so 113 years ago. Now this was an incredibly influential book and also central for the emergence of psychoanalysis and has been used extensively to interpret quotes why we dream.
Now the prime, in very summary fashion, the prime idea that Freud expressed is that dreaming is equivalent to wish-fulling your dreams, your wishes, to fulfilling your wishes. So that's why it's called wish fulfillment dreams.
Now, that's interesting because one of the stories he has in that book of his, Interpretation Of Dreams, is a woman he was psychoanalyzing who one day when she came to be, to her session said you know you told me the other day that dreams are wish fulfillment's. She said, I don't believe that, I had a dream last night and it didn't go along with wish fulfillment and Freud said, I want you to tell me what the dream was about.
Well, my dream was that I went to a store to buy some food because I was going to have a dinner party and when I got to the store it was closed and I could, as much as I wanted to I couldn't buy the food for the dinner. Freud scratched his eyes, you know I'm making that up, and he said that's true, he said you know what?
You didn't want to give a dinner party that's why you dreamt that. So that's famous psychoanalytic stuff. You can always twist things around so that it fits with your hypotheses, in this case Freud felt that indeed even though she had this, what she thought was a contrary dream it was a wish fulfillment dream.
Well that's one part of dreaming, what the other part that Freud had emphasized is that when we dream so many of our wishes are actually unacceptable to ourselves and therefore we dream it at night and so he constructed in many other studies the idea that in humans we have three subdivisions of the mind.
We have the Id, the ego and the super ego, you all know that right? So what it means that when you dream at night some of the wishes that you, unacceptable wishes that aren't in your Id, kind of seep through because the super ego is not under control since you're asleep. So that was his basic idea and of course it was then many years later discovered that whenever you dream you make all kinds of eye movements. You don't make eye movements when you don't dream but when you dream you make eye movements.
Now one of the problems with the Freudian theory is that animals also dream and in fact most dramatically, animals that hibernate do a lot of dreaming and not only dreaming but those animals also move their eyes about a lot OK? So that observation then kind of shifted the notion as to why we have REM sleep and so people thought about that and one observation that had been made is that when you eliminate a persons ability to move the eyes, such as you lose somehow the ability to activate your eye muscles, this can be done also in monkeys.
What happens in fairly short order is it your eye becomes ill-affected and what I mean by that is that the eye loses it's perfect roundness to some degree and more notably even what happens is that your cornea becomes uneven, becomes ridged, because you're not moving your eye.
So that discovery then has led to an alternate theory about why we dream which is not nearly as romantic or intriguing as Freudian theory, namely that we have REM sleep at night in order-- and especially have it in animals that hibernate-- in order to keep the eyes in healthy condition and to keep the cornea nice and smooth and even because if you were not to dream at all and you would sleep eight or 10 hours.
Then you would have an uneven, would result in having an uneven cornea that would make it more difficult for you to see and the reason for this then is that animals that, which presumably don't have Ids, egos, and super egos also dream as do animals that hibernate and so there's a necessity to move your eyes while you are sleeping or hibernating.
So that's an alternate theory and we'll see one of these years whether they're correct but I can tell you, which may not be very nice, that basically Freudian theory has taken quite a nose dive and in fact today psychoanalysis has become largely dead for a number of reasons, and so you don't have too many psychiatrists or psychoanalysts out there performing those psychoanalytic tasks that they had, in which a patient lies down on a couch and sort of free associates and sometimes even gets hypnotized to talk about some of his unconscious wishes and so on.
So anyway that's the story for today and that brings me to an end to eye movement control. I hope you'll appreciate the fact that even a simple system like eye movements is unbelievably complex when it comes to the brain controlling it.
Next time we're going to talk about eye movements and towards the end of that I'll come back a little bit and talk more about yet another aspect of eye movement control. Does anybody have any questions? Yes please.
AUDIENCE: Can you just explain again quickly why the bicuculline injection in V1 causes interference?
PROFESSOR: OK, that's a very good question. Why does bicuculline in the, in V1 cause interference? Because it screws up the neurons ability to analyze the visual scene. You mess up the centers around antagonism, you mess up the orientation selectivity of these cells, or direction selectivity of them, so they're no longer able to analyze the visual scene in the normal fashion. Yeah that's, and, it certainly you see, the V1 is quite far removed really from the generation of a motor response.
If you inject GABA inhibitors into areas which are closely linked to the execution of motor acts then the, the effect is seen because you generate a motor response or if it's muscimol then you inhibit the motor response but the frontal, you see this in the frontal eye fields but you don't see this in V1 because V1 is predominantly the system that analyzes visual percepts, as are V2 and V4 and all those other higher cortical visual areas.
Any further questions? All right very good so I will see you then next Monday and I think you'll find that we'll have an interesting session. We're going talk about movement and we're not going talk about regular movement, we're also going to talk about confusing kinds of movements, like especially very important apparent motion. You realize and just to say one more word here, that nowadays when you go home and watch television almost all the motion that you see on the TV is apparent motion, not real motion and I-- keep thinking about that-- and I'll tell you about that next time.
AUDIENCE: Monday is actually a Holiday.
PROFESSOR: Oh, yeah. Sorry it's not Monday. It's our next session is next Wednesday. Sorry about that.
Free Downloads
Video
- iTunes U (MP4 - 150MB)
- Internet Archive (MP4 - 150MB)
Subtitle
- English - US (SRT)