Flash and JavaScript are required for this feature.
Download the video from iTunes U or the Internet Archive.
Description: This lecture covers the auditory brainstem reflexes and descending systems, including the olivocochlear neurons and the middle ear muscles.
Instructor: Chris Brown
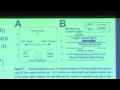
Lec 19: Descending systems ...
The following content is provided under a Creative Commons license. Your support will help MIT OpenCourseWare continue to offer high-quality educational resources for free. To make a donation, or to view additional materials from hundreds of MIT courses, visit MIT OpenCourseWare at ocw.mit.edu.
PROFESSOR: OK, I guess we'll get started. So last time we talked about hearing loss, and deafness, and cochlear implants. Any questions?
So did anyone read the Sunday New York Times yesterday? My email really lit up when this front page article showed up and it was titled, "Ground Shaking Noise Rocks NFL and Eardrums Take a Big Hit."
So the reason my email lit up is because my lab director is quoted. So it's a big deal to be quoted in the Sunday Times, right?
So this is talking about the NFL games where they have a noise meter down on the field and they encourage the fans to make a lot of noise. And apparently at the Seattle Seahawks recent game-- whatever-- the crowd was recorded at making 136 decibel noise in September. And so it says later on in the article, "Fans accustomed to hollering may--" is that what they do at the Seahawks games? "May scoff at the warnings as nanny state silliness. But to auditory experts, the danger is very real. People think it's cool or funny, or whatever. But there is increasing--" this is a quote "Increasing evidence that if your ears are ringing, damage is happening, said M. Charles Liberman, a professor of otology at Harvard Medical School, and the director of a hearing research lab at the Massachusetts Eye And Ear Infirmary." So he's a director of the lab where we're going to have a lab tour later.
"There's something irreversible going on, and it's only going to get worse as you get older. Liberman's research shows that even in the immediate effects of noise exposure after the immediate effects subside-- the ringing, the muffling, the feeling of pressure-- ears do not really recover."
So noise damage in the news. I'll pass it around. All right. So yeah, question?
AUDIENCE: I just have a quick question. So when I'm down at the T, it's extremely loud. Do you happen to know how loud that is in decibels?
PROFESSOR: I've never measured it on the T.
AUDIENCE: I had a friend who tried. And the thing on his phone wouldn't go high enough.
PROFESSOR: Yes. And a lot of folks have a sound level meter app. So how high was the maximum?
AUDIENCE: The max was 100, and it more than maxed out.
PROFESSOR: Yeah. Well, so I wouldn't be surprised if it's 110 dB on the T. Certain in between stops runs are louder than others as you know if you ride the T. So I think that that's not so damaging that it would hurt your hearing. But if you were the conductor or the driver on the train, you really have to worry about it.
On the other hand, if you're the driver, you have to have good hearing to respond to some kind of signals. So it's not like they can just wear hearing protection.
In this article about NFL fans, they said in some games they hand out those little foam ear plugs, which attenuate the noise as much as 20 dB. So if you're listening to 120, put the noise-- ear plug in and it goes down to 100.
But it also said that some people, like children, can't fit the foam ear plugs in their ear canal. So it doesn't work for them. And some people, like people who work on the T, need good hearing.
So today, we're going to move on and get back to the auditory brainstem. We had talked about the auditory pathway that comes up from the cochlea through the auditory nerve and into the cochlear nucleus, which is the very lowest level of the brainstem. And now we're going to talk about some higher levels of the brainstem. And especially some pathways that are called descending systems and brainstem reflexes. So we'll define what those two things are.
We'll talk especially about one descending system, which is a brainstem reflex called the olivocochlear neurons. Their anatomy, their functions, and their reflex pathway. And let me just stop and tie in once again with hearing loss.
These two brainstem reflexes that I'm going to talk about clearly protect the ear from damage due to high-level sound. So that is certainly one of the functions of the Olivocochlear neurons. And we'll see how that takes place.
The second brain stem reflex that we'll talk about is the middle ear muscle reflex. There are two muscles in your middle ear. When they contract, they make your sense of hearing less sensitive. Why would you ever want that to happen?
Well again, these muscles contract when you're in a high-level sound environment, like at the NFL game. And one of their functions is to reduce the sound getting into your inner ear. So to prevent damage from the high-level sound that might otherwise damage your hair cells. So that's the subject of today's talk. Now, what do I mean by reflexes and descending systems?
Well, this is a nice diagram from Michael Slama, who shows in the solid lines the pathways going up, which we've been talking about. And sometimes those pathways are called ascending systems because they go up. So it says here in red, the solid lines are ascending pathways. So by ascending, we mean from a lower level up to a higher. And ultimately, to the highest level in the neural pathway to the cortex.
There are analogous descending systems, which are shown here in dashed lines. And I think you can see some of them. For example, here's auditory cortex. And there's some dashed lines, which means cell bodies sitting an auditory cortex that project their axons down and end at the next lowest level, which is the auditory thalamus or the medial geniculate. And so that would be an example of a descending system because the information is starting at the higher level and going down to a lower level.
And at every junction between nuclei you can find descending systems. And in the auditory pathway, even at the lowest level, you have a descending system that starts here in the superior olive and goes all the way out to the cochlea. And we talked about some efferent nerve endings on the hair cells and the nerve fibers. And that descending system is called the olivocochlear system of neurons because it starts out in the olive and goes to the cochlear. OK, so that's what descending systems is, starting from higher levels and going down to lower levels. Now, how does that work out with brainstem reflexes?
Well, we've said that one of the functions of these olivocochlear neurons would be they prevent the cochlea from being damaged by high-level sounds. Well, when would you activate that system?
Well, obviously, when you hear a loud sound. So the ascending pathway has to come into the brain, synapse in the cochlear nucleus, go up to the olivary complex, and then come back down to the cochlear to prevent the damage. And so that little loop that I just diagrammed could be called a reflex pathway. So let's define what a reflex is.
What is a reflex? Anybody know? What does it mean to act reflexively?
AUDIENCE: Automatic reaction.
PROFESSOR: Right. So an automatic response. And certainly, these olivocochlear neurons turn on automatically. And sort of in between the lines of that definition means that the reflex pathway is operating down here in the automatic portion of your auditory pathway in the brainstem. The part where you think the auditory cortex or the other regions of cortex doesn't have to get involved. You don't have to say, OK, I'm in a loud environment. Should I respond with this reflex?
OK. Yeah, maybe I will. OK, respond. You don't have to think about it. It's automatic because it happens in the parts of the brain where things happen automatically. What other things happen in the brainstem?
Well, control of breathing. You have the motor neurons that come to your chest muscles and your diaphragm that enable you to breathe. Those motor neurons are located in the brainstem. And the control of respiration is this brainstem function. It doesn't have to go all the way to the cortex and have to think about it.
Now, that's not to say that reflexes, like the breathing reflex, you can say, OK, I'm going to stop breathing for a minute. OK, I'm going to hold my breath. So you can, via your higher centers, say I'm going to send information down here. Maybe some of these other descending pathways come down to the olivocochlear neurons and say, I'm not going to do this reflex for a moment.
Of course, you eventually have to start breathing again. But you can have higher center control of these reflexes. It's not that the higher centers aren't involved.
OK, so another reflex would be your patellar tendon reflex. The physician tests when you go to your doctor's office. They hit your patellar tendon with a little mallet and see that your leg contracts.
And apparently, everyone passes that test, right? You can't walk into the doctor's office without having some kind of reflex. But they're actually testing for hyper-reflex in that case. And so some of these reflexes can be in overdrive. And that's what the physician is testing for.
OK, so we are really interested in what all these descending systems do. What do the descending systems do in general?
Well, it actually ends up being a very hard issue to study. We know that those systems are there, but what good are they? What do they do in our sense of hearing?
So the classic, old-fashioned way of studying such a system would be to go in and make a lesion and see how the animal's behavior, the person's behavior changes, or do some kind of test. But it turns out most of these descending systems are intertwined with their corresponding ascending pathway. So it's very difficult to go in and make a cut or a burning lesion that just lesions one of the descending pathways and not affect the ascending pathway, which would complicate the interpretation.
The reason that some of these systems-- for example, the olivocochlear neurons-- are amenable and we know a lot about them-- are amenable to experimentation-- is we can selectively lesion and stimulate them in isolation from the ascending systems. And let me show you a diagram done of the olivocochlear system that illustrates that.
So these are the olivocochlear neurons, these stars here. There are two types. The red are the so-called medial olivocochlear neurons because they're sitting in the medial part of the superior olive. The green ones are so-called lateral olivocochlear neurons because they're sitting in the more lateral part the superior olive.
And this diagram shows the cell bodies of those olivocochlear neurons that are innovating this cochlea-- sending axons out to this cochlea on the right side. And that defines this cochlea as the ipsilateral cochlea. Ipsi means the same. So that cochlea gets a name. That's the ipsilateral cochlear.
And you can see that these green lateral olivocochlear neurons are basically on the same side of the brain stem as the cochlea that they innervate. But these medial olivocochlear neurons are distributed on both sides of the brain. So there's a little bit difference in anatomy.
Now, these are a little bit like neurons that we call motor neurons. Everybody knows what a motor neuron is, right?
It's going from the brain of the spinal cord out to the muscle. That's why it's called a motor neuron. And when it fires off, it contracts the muscle that it's innervating. It turns out all the muscles on the side of the body, let's say the ipsilateral muscles, the motor neurons are located on that same side of the brain. So right-side muscles are always innervated by right-side motor neurons. So this anatomy is a little bit different than the anatomy of motor neurons. Even though these neurons are having an effect in the cochlea, they're not innervating muscles. They're innervating hair cells in this case.
They do use the same neurotransmitter as muscles. So the olivocochlear neurons use the neurotransmitter acetylcholine. And so they would be called cholinergic.
OK, so they synthesize the acetylcholine and their cell bodies are transported down to the axons and the nerve terminals. Out in the cochlea use acetylcholine. They release it. Now, where do they release it to?
They release it to their targets. And out in the periphery, these Medial Olivocochlear Neurons, or the MOC neurons, target the outer hair cells. And they release acetylcholine directly onto the outer hair cells via their synapses.
The lateral olivocochlear neurons come out to the periphery and they release the acetylcholine in their synapses on the auditory nerve fiber peripheral dendrites. OK, so the innervation is very distinct. What are the auditory nerve peripheral dendrites?
Well, those are the ones we've been talking about. We've been talking about auditory nerve fibers. They start at the inner hair cells for the most part. They send messages. That's what this arrow is indicating. Send messages into the brain.
When there's a sound, the membranes move. The outer hair cells are electromotile. The inner hair cells respond. They send messages, synaptic messages, to their nerve fibers. The nerve fibers spike and they send information to the brain through the cochlear nucleus.
These efferent fibers are sending messages the opposite way. They're starting in the brain and going out to the cochlea. And out in the cochlea, this arrow means that the information is coming from the brain out to the outer hair cells or the auditory nerve dendrites here. Is everybody clear about that?
Now, the anatomy works out so that you can do some pretty interesting things. You can make a cut of this nerve bundle, the olivocochlear nerve bundle. You can stimulate it.
And right there underneath where the word "brainstem" is, there's a great place to make a cut or to electrically stimulate and activate this system. Well, why don't we use sound to stimulate the system?
Well, it's a little messier. It's cleaner to activate this bundle with electrical stimulation because you can put your stimulating electron right on it and only activate that system. So that's one big advantage. You can selectively activate these olivocochlear neurons and then study, well, what the heck do they do out in the cochlea? What changes do they have when you activate them? And what changes happen when you deactivate them and making a cut in the system? And de-efferent, if you will, the cochlea.
And as this list shows, there are a number of functions. And if I had to pick one of these, each of these functions has some experimental support. And I don't think I would be able to pick which is the most important.
Well, sure, if you're at an NFL game, you are probably experiencing a very high sound level. And so it becomes important in that situation to protect the cochlea from damage. So it depends on the situation. But we're going to go through these.
In turn, I think that since I mentioned damage, and since I don't have a slide on it, let me say how that experimental evidence arises. It's a very simple type of experiment.
You take an animal. You put it in a high-level sound environment comparable to an NFL game, 120 dB. Take the animal out, you study its cochleas. You can count the hair cells. You can measure the responses.
The hearing has become much less sensitive. Some of the hair cells have been killed. OK, no big deal. We went over that last time.
Take a second animal. And in that animal, cut this olivocochlear bundle going out to, let's say, the ipsilateral cochlea. The olivocochlear neurons going to this other side, the contralateral cochlea, leave that intact. OK, beautiful experiment because within just one animal, you have one side's cochlea has been de-efferented. You have cut off these efferent fibers. The other side has a normal innervation.
Expose the animal to 120 dB SPL. Do the same response metrics. Test the responses of the cochlea. Look at how many hair cells have been killed.
You find that there is a huge difference in the two ears. Where the efference or these olivocochlear neurons have been cut, there's a lot of damage and a lot of loss of sensitivity. On the intact side, it has been protected. There is less damage and better responses. It's more sensitive. So it's a very, very nice, very elegant experiment that's been done many times.
In probably both of these systems, the medials and the laterals provide such protection from damage. So we can say one of the functions then is then protection.
What do I mean by these other functions-- shift the dynamic range of hearing, reduce the effects of noise masking, and reduce hearing sensitivity when paying attention to visual tasks? OK, we're going to go over those one by one.
For the first one, the experiment runs like this. You record from these afferent auditory nerve fibers. And instead of cutting these olivocochlear neurons, you stimulate them. You can put a stimulating electrode right down there, right below the word "brainstem," and activate this bundle. What happens to the responses of the nerve fibers when you activate this olivocochlear system?
And that's what's shown here. And this experiment has been done since the 1970s. This is an old experiment.
This is the response in terms of the firing rate from the auditory nerve. And in this case, a tone is on. So you're really driving the auditory nerve fiber. So the firing rate is high.
Then, during the second black bar here, you stimulate the olivocochlear neurons that are going out to the cochlea. And look what happens to the firing rate. It goes almost down to zero.
When you turn that stimulation off, the firing rate comes back to about what it was before. There's been a huge inhibition of firing rate in the auditory nerve.
If you plot the firing rate as a function of the tone burst level-- so now we're going to do different tones at different levels. At low tone levels where the fiber is firing spontaneously, there's hardly any effect. In mid-levels, like we saw illustrated here, this is without stimulation. This is with stimulation. There's a huge decrease in firing rate. And up at the highest levels where the fiber has become saturated-- saturation means that even though you're increasing the tone burst, you're not increasing the fire rate coming from the fiber-- there's very little effect of this stimulation. How can that help us?
We have a dynamic range problem in hearing. Most auditory nerve fibers-- forget about the stimulation right now. Look at the solid curve. The dynamic range of most auditory nerve fibers has just 20 or 30 dB before saturating. That means the fiber goes up and it saturates. What happens when we get to the NFL game or in the better case, what happens when we're in a restaurant or a bar and we want to listen to the speaker across the table from us?
Well, that's a pretty high sound level, 80 dB. It's not damaging, but it's high enough to saturate our auditory nerve fibers. That means when the added sound level of the person across the table from you, their voice, adds to the background, they're not going to change the firing rate of your auditory nerve fibers. And the brain won't know except by seeing the person's lips move that there is speech.
It's much better to understand any kind of signals to be within this rising function of the firing rate curve, not in the saturated function where there's no change in firing rate. OK, so how can the olivocochlear system help us with this dynamic range problem?
Well, I've been emphasizing the decrease of firing rates. But as you can see from this curve with stimulation of the OC neurons, the effect is to shift this function over. In this case, it's about a 20 dB shift.
And now, at 70 dB, you're in the dynamic range of the function. And you're not saturated anymore as you were before. So one of the functions, we think then from this type of experiment, is the MOC efference kick in, reflexively kick in when you're in a high-level sound environment. And they shift the firing rate functions over so you can now understand a speaker's voice in a high-level background.
Now, there are some other factors that change an increase of dynamic range of hearing, which I don't think are important to the course. But we have talked about two-tone suppression. This certainly does the same kind of thing.
We're going to talk about contraction of the middle ear muscles, which also helps you with the dynamic range problem. So this is clearly then, one important function of the olivocochlear neurons. That is, shifting dynamic range.
Now, is there experimental evidence besides what you see here looking at firing rates of auditory nerve fibers to suggest that the olivocochlear system really does this?
There is a little bit. That is, with animals trained to detect changes in tones in a background of noise, they do it a little bit better when they have an intact olivocochlear system.
You can't do these experiments in humans because it's very difficult to turn this system off. We don't know a way of turning it off or interrupting it. And people probably call this system into play reflexively. So it's difficult to do these kinds of experiments in humans.
Now, there's another important function of the olivocochlear system, which is probably to reduce the effects of noise masking. And we haven't talked too much about masking in this course.
I mean, two-tone suppression is a kind of masking. Masking is where you're listening to one sound and a second sound comes in and interferes your ability to detect that very first sound. So I have a demonstration, though, that I think will convince you that masking is very important. And it runs like this.
So the demonstration is going to be you're listening to tone bursts, which are the pink things here. And they're at 2,000 Hertz, so it's going to be kind of a little bit above our 1,000 standard middle-of-the-hearing-range frequency. And it's going to give you 10 tone bursts and they're each going to be successively softer in sound level. And you're supposed to count how many steps you can hear. And I think there are 10 of them. We should be able to hear all 10 with the way I have set the level.
The second part of the demo is now the signal is masked with broadband noise. So the broadband noise will come on first. Shh. And these tone pips will be on top of that noise. And you're supposed to count now how many tone pips you can hear with the broadband noise.
And I think there's a little verbiage before this. Just ignore that.
[AUDIO PLAYBACK]
-Critical bands by masking. You will hear a 2,000 Hertz tone in 10 decreasing steps of 5 decibels. Count how many steps you can hear. Series are presented twice.
[BEEPING]
[END AUDIO PLAYBACK]
PROFESSOR: OK, so could everybody hear most of them? 10? All 10? OK.
Here's the masked.
[AUDIO PLAYBACK]
-Now the signal is masked with broadband noise.
[BEEPING]
[END AUDIO PLAYBACK]
PROFESSOR: OK, how many now?
AUDIENCE: Five.
PROFESSOR: Five? I mean, this is not a bad illustration, right?
When the pink tone bursts get within the black noise, they're pretty much disappearing. So clearly the noise was an effective masker of the tone pip.
Now, we had the example earlier of the phenomenon called two-tone suppression. And that was measured in an auditory nerve fiber. And everybody should be able to draw an auditory nerve fiber tuning curve.
On the x-axis, we have the frequency of the sound in kilohertz. And the y-axis, we have the sound pressure level for a response. An auditory nerve tuning curves, at least those with high CFs, look like this. And the characteristic frequency is the frequency right here.
In two-tone suppression, what we had is two tones. The first tone is a probe tone, which is placed inside the nerve fibers response area. And if you look at a graph of firing rate-- so this is firing-- and you turn that tone on, the firing rate is going to go way up. Maybe 100 spikes per second because it's within the response area.
Now, the second tone, sometimes called the suppressor, is put outside the response area but close to it. OK, so here's the probe. This is the probe tone, high firing rate. And now a little bit later, we're going to turn on a second tone. And this is called the suppressor. And I haven't drawn this very well, but when the suppressor goes on, the firing rate can come back down.
This goes off first. And come back up. OK. So clearly, at least in the discharges of auditory nerve fibers, you can have suppressors outside the response areas that decrease the response to probes.
This probe tone is going to be signaled by auditory nerve fibers close to that frequency. So many auditory nerve fibers have CFs close to 2 kilohertz.
This noise has energy throughout the frequency range, if it's absolutely white noise. Some of its noise will be within the response area, but some of it will be in suppression areas. And these suppression areas can be either side of the excitatory area. And they can be big.
And in some cases, they can overrule the excitation, or at least decrease it. So some of the reason you couldn't hear the tone when it was masked by the noise is because of two-tone suppression. This is sometimes called suppressive masking.
There's another kind of masking that's important-- it may be equally important-- and it's called adaptive masking. And it comes from the process called adaptation.
Almost all sensory systems have adaptation, which means that when you turn a stimulus on, you get a vigorous response. And even though the stimulus stays on, after a while the response dies down a little bit. And that process is called adaptation. So I've illustrated the process of adaptation for auditory nerve fibers in this next graph.
Here's that pink tone burst at 2,000 Hertz we were listening to. There's the auditory nerve response to it. Right as the tone burst goes on, there's going to be a vigorous discharge, which die eyes down and becomes a smaller-- still a discharge, but a smaller discharge. And that process is called adaptation.
Where do you think that process arises? OK, while you're thinking about that, I'm going to draw a picture of what's happening here.
So you have the three rows of outer hair cells. We have the inner hair cell. We have the auditory nerve fiber. And we're recording here. And we're saying, we turn the sound on and you get a whole bunch of spikes from that single auditory nerve fiber.
But after a few milliseconds or so, the response dies down. What experiment could you do? We don't know where that process is arising. We can't explain it. What experiment would you do to study where that comes from? Anybody?
Here's the tone. Here's the response from the nerve fiber. What do we need to figure out where that adaptation is taking place? Well, how about recording from somewhere else? What about recording from the hair cell?
OK, if you do that, the hair cell doesn't fire spikes, but it has a receptor potential in response to the sound. The receptor goes on and stays on. OK, where is adaptation taking place?
Well, somewhere between the hair cell and the nerve fiber. The hair cell doesn't adapt, the nerve fiber does adapt. what could explain adaptation then given that? Anybody? OK, what do we have here?
We have a synaptic ribbon with lots of synaptic vesicles. The tone goes on. You have a whole bunch of synaptic vesicles. You release them because the hair cell has depolarized. You have a burst of auditory nerve firing. And you can make new synaptic vesicles, right?
Yeah, but it takes time. OK, so as time goes on, you've released all of these or many of them. And you can make some new ones, but maybe not quite as fast as you've released them. So you deplete your synaptic vesicles. The hair cell's still responding. There are fewer vesicles and fewer neurotransmitter released to the nerve and so the nerve firing dies out.
So adaptation is often ascribed to the diminished release of neurotransmitter. And so far, that's all adaptation to a single tone. How does that explain masking?
Well, if your nerve fiber responded to the noise-- at the very beginning you heard that noise come on-- shh. And then the tone came on a little bit later. If the tone is high in level, sure, it's going to still have some synaptic vesicles to release. But if the tone is very soft, the hair cell is not going to be able to release synaptic vesicles because they've already been released at the beginning of the noise.
So adaptive masking is where you have, to a certain extent, run out of hair cell neurotransmitter. And there's none, or much less, left to release.
Clearly, adaptive masking means that this fiber is also responding to the mask or the noise in this case. So in adaptive masking you have to have a stimulus that excites. The masker has to excite the nerve fiber and the hair cell. And the probe-- probe is always exciting it. So that is a second explanation for masking.
Now in this case, the olivocochlear system can actually help you with adaptive masking. How can it help you?
Well, in this case, if you also-- when you start to hear this noise, you call that olivocochlear system into play. And it acts. What does it act to do?
It decreases the firing of the auditory nerve. Then when you have the tone come along, you have plenty of neurotransmitter left in the hair cell because you haven't released it all. And you have at least some to be released in response to the tone.
So in this case, the third function then of the olivocochlear system is to reduce the effects of masking. And we should have said back here when I listed the functions, reduce the effects of noise masking, especially adaptive noise masking. The kind where the masker excites the fiber unlike suppressive masking where the masker reduces the response.
There are certainly important and reliable studies where animals in which the olivocochlear bundle has been cut have much more of a problem with noise masking. They cannot detect signals that are buried in a noise masker as well as animals with an intact olivocochlear bundle. So clearly, that is a viable function.
Now finally, and this is pretty important for our course here, there's been this fourth idea of what the olivocochlear reflex could do. And it's always been a little bit wishy-washy because there hasn't been really good experimental evidence. Until this paper-- this paper Delano et al, 2007-- is the paper that we have listed for reading today.
And this paper clearly shows that another function of the olivocochlear system is when you're paying attention to something that is not auditory, like a visual stimulus, the olivocochlear system acts and reduces your sense of hearing. You're not using hearing for whatever task, so you desensitize your hearing and you pay attention to the visual system. OK, so how is this going to work?
So this paper trained chinchillas as the experimental animal. And the task was to pay attention to lights. So let me get my pointer here so I can point out a little better.
So this is the task in part A here. And this neutral cue is a light that's straight ahead from the experimental animal. And that goes on and says to the animal that the trial is starting. Then, that goes off and one of two targets appears. Either a left target or a right target. And these are little spots of light to the animal's left or to the right.
The task then is if the left target went on, the animal goes and presses the left lever. And if it does that correctly, it gets a food reward. If the light went on on the right, the right target was illuminated, the right lever is supposed to be pressed. And if the animal does that correctly, then it gets a food reward. If it does nothing or if it presses the wrong lever, it's punished by a timeout. And the animals are food deprived, so they're motivated to do this task. That's the visual task.
Now, on top of that there's some auditory stimuli presented. And they're not relevant to the task. They're just ongoing all the time. And here then in B are the target and the neutral cue lights and the response period. And the auditory stimulus going on all the time is a click or a tone burst. And it's just going on all the time. And they're making some recordings from the auditory system.
And in this case, they're making a recording from the round window of the cochlea. And if you had a microscope, you could see some little blips right here in response to the clicks. And they're pretty big here. But as time goes on, they get smaller.
And that's plotted right here. The response they're measuring is called the CAP, and that's the Compound Action Potential. And if you are astute here, the action potential is another word for "spikes" or "impulses." But they're not measuring from one single fiber, they're putting a big electrode on the round window of the cochlear and they're measuring the summed or compound action potential from the whole auditory nerve.
It's just a convenient place to do it. You can do it in an awake animal. You can do it in awake behaving animal like we have here.
When you turn on a click, almost all the auditory nerve fibers fire synchronously and you get a big response. Hardly have to do any averaging at all. OK, so that's the response, the compound action potential.
And this graph plots the CAP amplitude-- how big it is. Upward on the graph is a big amplitude and lower is a diminished amplitude. And right here at zero-- they just call it zero because that's sort of the baseline before the trial even starts.
So in this first bar here is the neutral OK cue. That's the thing in the middle that says to the animal, the task is starting. And right away during that neutral cue, these black dots show you that the compound action potential is decreasing.
Then, the neutral cue goes off and the target-- one of the targets goes on. Either the left or right. And the compound action potential further decreases. Then, the animal makes its response here in this dashed line. OK, gets its food reward and the CAP comes back up. OK, now how do we explain this?
Well, the CAP is the summed firing rate of many auditory nerve fibers. Instead of stimulation of the OC neurons decreasing the firing rate, essentially the animal itself has turned on its OC neurons and the firing rate has gone down. Or the summed response, in this case, has gone down.
The animal has said to the olivocochlear system, I'm starting a visual task. I don't want to pay attention to extraneous things, like sounds. So I'm going to decrease my sensitivity in hearing by activating these olivocochlear neurons. Now, pay attention to the important targets, which are visual.
Now, there are some other symbols in here that are open. They're a little bit harder to see. But there are some CAP amplitudes from another trial of these same animals. And for one reason or another, the chinchillas didn't always do the task. Every now and then they would just not pay attention to it. They wouldn't press the lever. They wouldn't respond at all. As if they were sort of spaced out, thinking about something else if you will.
And in those cases, which are timeout trials. Let's see, what did they call them?
Ah, omissions. This little symbol right here is omissions. So it's an omission trial where the animal didn't even do the task. In that case, the olivocochlear system is apparently not called into play at all. The animal is just not doing the task.
Now, the investigators were very astute and they said, we're going to make the task a little more difficult for the animals and see if we get a bigger effect here. And the way to make the task difficult is make the targets brief.
So instead of the target going on for a couple of seconds, the target went on for just a half a second. And here's a trial with just a half a second. And there was a big decrease in the auditory response.
If the target went on for a long time, there was less of a decrease. And this is the effect plotted as a function of target duration. Brief targets made this job harder to do for the chinchillas.
So this is clearly, at least to me, some evidence that you call into play the olivocochlear system when you're doing a visual task. So maybe as humans, we do this when we're trying to read. We're concentrating on the book, visual stimulus. And our neighbors music is going on. It's not relevant to what we're doing. Maybe we're listening to it subconsciously, but we decrease the response to that auditory stimulus because it's not important to the task. So clearly then, this is good evidence from this single study for the last function for the olivocochlear system, which is that you reduce your hearing sensitivity when attending to visual or perhaps other modality tasks. So these are the four basic functions then for the olivocochlear system.
Now, before I leave the olivocochlear system, let's do a little review here on exactly how it's acting. So we said we're activating these systems going out to the hair cells and we're reducing the responses of the auditory nerve fibers. Something I haven't told you but was observed maybe 20 years after the phenomenon was first discovered is the idea of which of these systems is being used here when we electrically stimulate the system and activated it.
It turns out that the medial olivocochlear neurons have big, fat myelinated axons. And you can maybe appreciate that from this drawing that these are very thick lines. The lateral olivocochlear neurons have very thin axons. And one effect of that besides how fast they conduct impulses is when you stimulate, for example, at this position here in the brainstem, it takes a huge amount of stimulating current to activate very thin nerve fibers like the lateral olivocochlear neurons.
If you apply a huge stimulating current here, the current spreads to everywhere, including the facial nerve, which causes the experimental animal to twitch. So that's almost never done. More moderate levels of stimulation current are applied here. And at those levels, the predominant system activating is the medial olivocochlear neurons. Those MOC neurons send their fibers out to the outer hair cells. And we say when they act on the outer hair cells, the responses of the nerve fibers diminish. As we review, we should be able to account for that.
OK, we are stimulating nerve fibers that go out to the outer hair cells here. And we're recording the responses from the auditory nerve fibers right here. Of course, these olivocochlear neurons are sending messages out to the periphery. And the auditory nerve fibers are sending messages into the brain.
Somebody explain to me what's happening here. What are we doing to the cochlea to cause these responses to go down when we apply this simulation? Anybody?
We're affecting the outer hair cells, right? What are the outer hair cells? The cochlea amplifier, right?
It turns out that releasing the acetylcholine on to the outer hair cells decreases their electromotility. So in effect, it discusses the gain of the cochlear amplifier. If the cochlea is less amplified, the inner hair cell stereocilia are going to be bent less. They're going to release less neurotransmitter to the associated auditory nerve fibers. And we're going to measure less of a response. That is how the olivocochlear neurons-- those that go to the outer hair cells-- affect the responses of the auditory nerve fiber. So this decrease in firing rate then is a manifestation of turning down the gain of the cochlear amplifier.
It was quite a mystery when this innervation was first worked out before the outer hair cells really were known to be the amplifier. How could stimulation of fibers going to the outer hair cells give you decreases in responses of fibers coming from the inner hair cells?
And now that the outer hair cells are clearly associated with the cochlear amplifier, it's clear. Now, what was the loss of sensitivity when the outer hair cells were completely gone or when their prestin was knocked out? Anybody? What was the loss of sensitivity? 40 to 60 dB, right?
So if you eliminated the cochlear amplifier, we had a loss of 40 to 60 dB. What kind of effect do we have from stimulation of the olivocochlear neurons?
Well, the length of this arrow when brought down to the x-axis shows you how much sensitivity is lost. You could overcome this by dialing in an increase of sound pressure level to make up for it. You could titrate the effect of decreasing the gain by increasing the sound stimulus. And the width of that arrow, the length of that arrow, is about 25 dB.
So instead of eliminating the cochlear amplifier, you just reduce its gain by the action of these olivocochlear neurons. The effect is about 25 dB. Which is a fairly strong effect, but you're not completely ridding the cochlea of the cochlear amplifier. You're decreasing its gain. OK, so that's kind of a review on outer hair cell function and how these olivocochlear neurons affect the outer hair cells.
So you really have a controllable cochlear amplifier controlled by these olivocochlear neurons, or efferent neurons, or descending neurons coming from the brain out to the cochlea. Any questions about that?
OK. If there aren't, then I'll go on to another reflex pathway. Maybe I actually do have one more thing to talk about. Right. Let me just mention this idea that this reflex pathway is kind of getting teased out by current studies. And it can be a little bit complicated, so let me show you what people are working on now in the olivocochlear reflex.
Here, I think I've flipped the slide on you so that this slide shows the MOC neurons going to the left cochlea. OK. That's the ipsilateral cochlea. How do these MOC neurons get their inputs? Where do they come from?
OK. Well, the red and blue are the olivocochlear neurons coming out to the cochlea and the purple are their inputs. And all of the inputs have to, of course, use the cochlea. To get this olivocochlear neurons to fire in response to sound, you have to activate the cochlea. You activate the auditory nerve. The auditory nerve goes into the cochlea nucleus then. And that's where the limits of our understanding are sort of-- we are approaching the limits of our understanding. What are the cochlear nucleus neurons that are the so-called MOC reflex interneurons that are drawn in purple here?
When we talked about the cochlear nucleus, we identified a bunch of different types of neurons. We had spherical cells, globular cells. Both of those are known as bushy cells. We had stellate cells. We had pyramidal cells. We had octopus cells. Which of those provide the inputs for the MOC neurons?
And that's assuming a direct pathway, which I'll give you from some other experimental evidence which I won't show you. At least one group of cochlear nucleus neurons projects to the MOC neurons. And how do I know that?
Well, you can record from the MOC neurons and turn a sound on. And in less than 5 milliseconds, you can get a response. So there isn't enough time for the reflex pathway to go up to the auditory cortex, which takes about 4 or 5 synapses. And to go around there and start coming down, that takes 10 or 20 milliseconds. You get a response here very quickly. And so there isn't enough time, except for cochlear nucleus to project directly there. So which of those cochlear nucleus neurons do project?
Well, we don't know absolutely for sure. But it looks like from lesion studies that probably the stellate cells in the cochlear nucleus. And especially the stellate cells in the part of the cochlear nucleus called the PVCN. And I think we talked about the ventral cochlear nucleus and the dorsal cochlear nucleus as being the big main divisions. In the VCN, there's two subdivisions, AVCN and PVCN. And the stellate cells that seem to be-- the MOC reflex interneurons are in the PVCN. How do we know that?
You can make lesion studies in various parts of the cochlear nucleus. If you lesion the DCN, this reflex goes along fine. If you lesion the AVCN, it goes along fine.
In the PVCN, if you make a lesion there, the reflex is interrupted. In the PVCN, there are a number of types of neurons. The ones that seem to have the right characteristics in terms of latency, sustainability of response, tuning, are the stellate cells. So it's a little bit of a squishy argument. Stellate cells seem to project to the right way. So most of the evidence is behind the idea that those particular cochlear nucleus cells are the ones that are the reflex interneurons.
Now, another point of this slide is that the MOC reflex pathway is consensually organize. So what does consensual mean? What does consensual in terms of reflex mean? Not in terms of consensual sex, in terms of reflexes or brainstem organization. How does the detective in the old 1950s black and white movie come to the victim whose lying down and shine a flashlight in the eye-- how does he decide that the person is really dead?
The pupil constricts if the person's still alive, right? The brainstem is still working. It's a very quick test. You don't have to have a stethoscope and listen to the heartbeat. You can be brain dead and still have a heartbeat. But if you shine light in a person's eye, the pupil constricts, right? So that is a brainstem reflects. The pupillary constriction reflex.
It turns out if you shine light in the right eye, what happens to your left pupil? It constricts, right.
So both pupils constrict from just a stimulus in one eye. And that is called consensual. It means in agreement. The left and the right side do the same thing in agreement. So most of these brainstem reflexes are consensually organized.
And it turns out that you can look at the MOC reflex in your left ear. And obviously, if you put sound in there, that reflex is going to take place. But you can also elicit that reflex by sound in the right ear.
For some reason, sound in either ear can activate the reflex. Not quite as well, labeling studies have shown that just some of the MOC neurons are responsive to sound in the ipsilateral cochlea. That is, the cochlea that they project to. And about half as many are responsive to sound in the other ear. The so-called contralateral cochlea and the so-called contra response, MOC neurons in red here.
Labeling studies have shown these contra response neurons are sitting on the side of the brain as the cochlea that they innervate. But the ipsi response neurons are located on the opposite side of the brain [INAUDIBLE]. And knowing those response characteristics, then you can draw the purple pathway that's necessary to drive them.
These contra response neurons respond to sound over here, so they must get this purple arrow. These ipsi response neurons are responsive to sound here, so they must get this purple pathway. And it's kind of complicated, but that's the kind of studies that people do now on these brainstem reflexes.
And I want to just say one more thing. People are now working on where the neurons go in the cochlea. So these are some tuning curves from these MOC neurons. So they show sharp tuning just like the auditory nerve. And where do they go?
Well, they have a very nice so-called tonotopic projection into the cochlea. That is, an MOC neuron with a high CF goes and innervates at a very basal cochlear location. and. An MOC neuron with a low CF goes and innervates closer to the apex.
And this black line shows the mapping for auditory nerve fibers. Those are the ones coming out of the cochlea. And the colored dots show the mapping for the fibers going back on to the cochlea.
Everything, remember, in the auditory system is tonotopically organized. So it's no surprise that that would happen. But it opens up the idea maybe that if you're interested in turning off your sense of hearing just for low frequencies, you could activate these fibers, go out to the cochlea in just the apical part, and leave the basal part. Let's say you're very interested in listening to high-frequency sounds. Leave the basal part intact. Or vice-versa.
You could control the cochlea in a frequency band by frequency band manner. The anatomical substrate there is laid for that type of manipulation. You don't have to shut down the whole sense of hearing.
OK, so let's move on to the second system that I want to talk about. That is, the brainstem reflexes associated with the stapedius and tenser tympani middle-ear muscles. These are two muscles that are in the middle ear. Remember the middle ear was the part of the ear that starts at the eardrum and ends at the cochlea?
There are two middle ear muscles. There's a tensor tympani. This is shaded in brown here. And there's the stapedius shaded in red here. The stapedius tugs on the stapes. And the tensor tympani tugs on the malleus. But it gets its name because when it contracts, it looks like-- if you're looking at the eardrum through an otoscope, it looks like the tympanic membrane gets really tense. It looks a little flaccid before it contracts, and then it gets real tense. So it's a tensor tympani. And what happens when those muscles contract?
That's shown in this next slide. They reduce the sound transmission through the middle ear. This is just simply a graph on contraction of the stapedius muscle. This is during the contraction. This is the magnitude change. So it looks like sound doesn't get through the middle ear anywhere near as well as normally. And you have about a 25 dB decrease in sensitivity.
Simply what that means is that usually the eardrum moves and these bones move and they convey efficiently the sound into the inner ear. But if you tug on the muscles and contract them, then the bones don't vibrate as easily. And the sound doesn't get into the inner ear. So this is again, like the olivocochlear system, an inhibitory system in that when it's activated it reduces your sensitivity of hearing. Now, how are these muscles controlled?
Well, they're controlled by neurons coming from the brain. But in the case of muscles, of course, they're always on the same side of the brain as the muscles they innervate. So the left stapedius muscle is controlled by motor neurons on the left side of your brain. And the stapedius muscle motor neurons run in the seventh cranial nerve. And what's the seventh cranial nerve? Anybody?
We have the eighth cranial nerve is auditory and vestibular. What's the seventh? Anybody?
AUDIENCE: Facial.
PROFESSOR: Facial, right. So the stapedius axons go in that cranial nerve. The stapedius motor neuron axons, should say.
And for the tensor tympani, it's the fifth cranial nerve. Tensor tympani. So some very interesting experiments have been done in people who have a compromise of their seventh cranial nerve. Does anybody know what Bell's palsy is? Does anybody know what a palsy is?
Palsy means your motor neurons aren't working so well. So what happens?
In Bell's palsy, the seventh cranial nerve innervates facial musculature. So if you have a problem, a cut seventh nerve or a Bell's palsy, which is a viral infection of the seventh cranial nerve on one side, one side of your facial features droop because the muscles there, which ordinary keep your muscles in good control of your face, they're not working anymore.
So a person with a Bell's palsy has a droopy face on that side. The other side is fine because the other seventh cranial nerve is working just fine. Or it usually is. It's very often the case that it's unilateral.
In Scandinavia where you can do experiments on humans, or you could at least do experiments on humans to a much greater extent than in the United States, what some enterprising researchers did was they took people who had Bell's palsy and they said, well, you can go and work in your ordinary job in the automobile factory where it's really loud. They tested their hearing before they went to work and they tested their hearing at the end of the work day. They tested it in the left ear and in the right ear. Let's say the right ear was the palsied ear. And then, left ear, which was normal, person came out at the end of the workday and their hearing was just right.
In the palsied side, at the end of the day they had a temporary threshold shift. That means that if they're hearing was down at 0 dB at the beginning of the day, their thresholds were elevated at the end of the day. And hopefully, it's temporary. That is, if you sleep all night and things recover, you're back to normal the next day.
But what had happened-- this is a beautiful experiment because it's controlled-- left-right in the same individual. So whatever that person did-- took drugs, or got infections, or whatever-- was hopefully bilateral. It's a human, so you can test very well thresholds of hearing.
Presumably then, the stapedius muscle, which was not working on the palsied side, couldn't protect the ear from the high levels of sound in the work environment. And there was damage-- hopefully, temporary-- to that person's hearing. So clearly, the contraction of these muscles, which makes sound not go through the middle ear as well, is of beneficial effect.
For example, they protect the cochlea from damage. People who are at the NFL games are having their middle ear muscles contract. Certainly, the stapedius. And probably the tensor tympani.
They probably reduce the effects of noise masking. So that is a little twist here in terms of the spectrum of where these muscles act. It turns out when the muscles contract, both the stapedius and the tensor tympani, they affect low frequencies to a much greater extent than highs.
For example, here you have a reduction in transmission through the middle ear of 25 dB at the lowest frequency and 0, or even actually, improved transmission at the high frequencies.
And if you've ever been in a car and you accelerate to go on the highway, you know the low rumbling of the sound of the car means you have to turn the radio up when you want to enjoy your music because the low rumbling of the car, these low frequencies tend to mask the interesting high frequencies or mid-frequencies of the music that you're trying to listen to on the radio. So low frequencies tend to mask mid and highs very effectively.
And so if you decrease the transmission for the low frequencies, you reduce the effects of noise masking. Especially by low-frequency noises.
Now finally, an interesting thing that is clearly known for the middle ear muscles is that they contract just before and during when you speak. During you speak, OK? I don't know who wrote that.
But anyway, when I speak, I'm contracting my middle ear muscles. And the idea there is perhaps when you're speaking, you don't want to listen to yourself. And if you're speaking in a loud voice, like 80 dB, I'm trying to project here. Or if I'm yelling at some family members, I want to not decrease my sense of hearing or damage it. So I contract my own middle ear muscles to prevent self-stimulation.
After I finish my speaking or vocalization, my muscles then stop their contraction, going back to normal. And then my sense of hearing is very acute because it hasn't been damaged by my own vocalization.
And later on in the course, when we talk about echolocation in bats. So bats send out this pulse of sound, which is their vocalization. And they listen for an echo. The pulse of sound can be 120 dB. They're really screaming because their targets, the insects that they hunt, are very, very small. And there's not much physical surface for that to reflect off. So the reflecting sound is very small. And so they maximize that reflection by emitting a very high-level acoustic pulse. And they don't want to damage or desensitize their hearing because they're listening to very soft reflections from the echoes. So bats clearly tense their middle ear muscles right before they make this echo-locating pulse.
We don't know if that's the case for the olivocochlear neurons. It just hasn't been investigated. It's very hard to measure their effects during vocalizations. But perhaps they do. Certainly, the middle ear muscles do.
OK, that's all I wanted to say so we're sort of out of time. Any questions?
And I want to make one announcement about Wednesday's class. So in Wednesday's class we're going to be talking about sound localization, and listening to sounds that differ in intraural timing and level difference. And so the usual demonstrations that I play aren't going to work very well because we want to manipulate just one of those cues. So we're going to be listening in headphones. So I have some great demos. So please, if you can, bring some headphones or earbuds. And maybe download the demos from the course website.
I have a few players that I can also circulate around. But if some people have these demos on their laptops, that would be more convenient. OK? See you on Wednesday.
Free Downloads
Video
- iTunes U (MP4 - 181MB)
- Internet Archive (MP4 - 181MB)
Subtitle
- English - US (SRT)