Flash and JavaScript are required for this feature.
Download the video from iTunes U or the Internet Archive.
Topics covered: EVA and Robotics on the Shuttle
Instructor: Prof. Hoffman
Subtitles are provided through the generous support of Heather Wood.
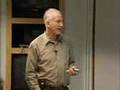
Lecture 20: EVA and Robotic...
Related Resources
Prof. Hoffman Bio (PDF)
There were a couple of areas that have been discussed a little bit, which I wanted to elaborate on because I think it is significant.
One of them relates to intact aborts.
The question of a crew escape system and whether that should be automated, whether the crew should have control and what is required if you actually have the crew take control.
I think Chris Kraft gave an interesting perspective with the idea that because of the difficulties, or given the requirements, really, the impossibility of incorporating a capsule escape system for the Shuttle, the project basically took the point of view that the Shuttle itself was the escape pod, which means that you have to recover the Shuttle intact regardless of any engine failures.
Now that, of course, requires 100% reliability of the solid rocket boosters which we never did achieve with Challenger.
Although, of course, had we operated it within its spec limits it might be another story.
But, in any case, I want to go through some of the details of a return to launch site abort and then I will describe an incident which occurred on our last flight to give you an idea of what is involved in the actual operation of abort modes.
The idea is you have the two solid rocket boosters which have to light.
And they are going to perform no matter what.
If one of those boosters doesn't light and the other does it is a bad day.
What can you say?
And, obviously, if one of them fails during flight it is a bad day.
And, which was more of a design problem, if they don't tail off at just the identical rate so that you get asymmetric thrust greater than a certain capability of the Shuttle to control, you have had a bad day.
And because of the requirement to have symmetric thrust, I don't think if this was discussed before, when the solid rocket boosters are poured, they mix a batch of propellant sort of like you mix bread dough.
And then they pour the propellant into both the left and the right booster segments at the same time so each booster segment has the identical batch of propellant in it.
It may not be the same from the bottom to the top, but it is the same from the left to the right.
And that is critical.
And then they always reserve a certain proportion of the propellant.
And they do tests on that to make sure that there are no process control problems.
OK.
You still need the three main engines to have enough thrust.
The boosters each give you about 2.5 million pounds thrust, so that is about five million pounds to get off the ground.
These give you about a half a million pound thrust at vacuum, four hundred and some odd thousand pounds thrust, so still over a million pounds thrust.
And, of course, the problem you have during first stage is you have big gravity losses.
The Shuttle on the pad weighs about 5.5 million pounds with all the propellant.
If you don't fire your main engines you are barely going to lift off the ground.
Actually, I guess it is a little bit less than five million pounds.
But, in any case, without the three main engines firing you don't have enough thrust to get into orbit.
Now, remember we talked about for deorbit burn, normally we fire both OMS engines.
If one of the OMS engines is out you can fire one engine for twice as long.
Or, if they are both out, you can fire the four RCS thrusters for twice as long again.
Because you are in orbit, you are weightless, you don't have gravity losses, so firing half as many engines for twice as long is completely equivalent.
When you are taking off against gravity that is not true.
I mean if you only have five million pounds of thrust for a five million pound payload you are just going to sit on the pad and burn all your fuel.
OK.
You need your three engines.
And, in fact, another interesting point, the attachment between the external tank and the Shuttle, that is stressed to assume that you have at least one engine burning.
They tell me that if the Shuttle took off with just the solid boosters and you didn't have any thrust, sort of keeping the Shuttle up with the external tank, that the structural attachment points would fail.
Anyway, you need your engines.
What happens if you lose an engine early on?
If you are far enough into the launch, you are over the main gravity loss segment usually starting at about 3.5 to 4 minutes into launch.
If you lose one engine you can make it over the ocean, And that is called a Trans-Atlantic Abort.
And, when you do that, you are basically flying upside down.
You will do a roll maneuver, drop your external tank and come in and land.
And you are basically going in the same direction which is nice when you are flying a rocket.
These things are hard to turn around.
But if you are going to do a return to launch site abort that is exactly what you have to do.
So, this is a procedure that has been analyzed to death in many, many computer simulations.
The basic procedure is you take off and you lose an engine.
Now, it doesn't matter when you lose an engine in the first 2.5 minutes.
You don't do anything while the solid rocket boosters are firing.
They basically have open loop guidance.
There is a certain projector programmed in and you cannot readjust that trajectory with a closed loop guidance solution to accommodate for any sort of a malfunction.
So, you don't even declare an RTLS abort.
And that, by the way, is done by the crew punching a button which essentially is a protected button.
You punch that.
And you can also do it via a computer input if something goes wrong with the button.
You declare an RTLS abort after SRB separation.
Immediately what happens is your trajectory increases because you want to gain altitude, and you will see why in a minute.
But what happens now is you've got a pretty hefty downrange velocity already and you have got to turn around and come back.
You have also got to worry about the disposal of your external tank.
If you turn around and you come back, you are still burning your engines, you are still riding your tank, and you don't want the tank coming down on Melbourne or Disney World or anything like that.
The basic procedure is as follows.
You are going out, you loft your trajectory a bit, once you get far enough out now what you do is you do a powered pitch around.
A very sporty maneuver.
The external tank is here.
Now you are flying backwards into your plume and everybody has speculated on what that would actually look like.
Now you are flying backwards decelerating.
And, as you decelerate, of course, gravity is starting to push you down so you've got to increase your pitch velocity until finally you basically have no more horizontal velocity and are just sitting statically on top of your exhaust.
And now you gradually pitch forward and you start to fly back.
Again, you have got to plan your trajectory so that when you drop the tank it is going to come down in the ocean.
And then, if all that goes well, now you enter the heading alignment circle.
And, of course, you are a heavy weight vehicle because you also, in the process of the RTLS, dump your OMS tanks and your RCS propellant so that you want to make sure you are as light as possible.
Plus, in case you have any problem with landing you don't want your hypergolic propellant tanks to rupture.
If all that goes well, now you enter the heading alignment circle and you have hopefully a nominal landing.
As I say, this has been analyzed in high-fidelity dynamic simulations.
The crew practices it over and over again.
Everybody knows the procedures.
Nobody wants to be the first crew to try this out.
Before STS-1, you remember we talked about how there were lots of problems with the tiles falling off.
I remember one of the people very high up in NASA, I don't remember if it was the administrator or one of the deputies, suggested that maybe the safest thing to do would be to do a planned RTLS because an RTLS, of course, you don't get up to those high velocities.
And so you don't have any thermal problems.
And so if a whole bunch of tiles had fallen off on the launch pad you could safely do an RTLS.
Well, obviously, they did not appreciate the difficulties of doing an RTLS and that suggestion was not taken up.
We basically decided we were not going to fly until we had confidence that the tiles were going to stay on.
Cut forward to February of 1996, which was my fifth and final Shuttle flight.
I was the second flight engineer so I was sitting right behind the pilot who is responsible for the main engine performance.
Now, I should tell you, we have had, in the course of the 114 Shuttle flights we have had four pad shutdowns, pad aborts.
And I think we talked about that briefly.
The main engines turn on six seconds before the solids.
Just reviewing that does two things.
It gives the engines time to come up to full thrust and then you can perform checks on them to make sure that they are operating properly before you commit yourself to flight.
And, of course, it gives time, because of the asymmetric thrust, for what we call the "twang" that the whole Shuttle stack goes forward and then back.
And then, when you get to the vertical, you light the boosters.
In fact, when they had the first test firing on the pad before STS-1, one of the reasons that they wanted to do that, in addition to just confirming that everything was working right, is they really wanted precise timing on the twang.
People had calculated it, but that is not something you want to get wrong.
So, they wanted an experimental verification.
Of the four pad shutdowns, two of them were caused by instrumentation and two of them were real engine problems.
I remember the first one was the last flight before what would have been my first flight back in 1984.
And the main engine cutoff is called MECO.
So, 8.5 minutes into the flight.
After they had a pad abort the flight engineer called to Mission Control we have MECO, somehow I thought we would be a little bit higher.
And, of course, when that happens now you've got to pull all the engines out, take them back to the shop, bring in new engines and it is about a two or three week stand down.
And the crew has to go back to Houston.
And, of course, all of your friends and family are there and they have to go home.
It is no fun.
I mean nobody complains if it is safe.
We're sitting on the pad, we're counting down and everything was on schedule.
In fact, the first of my five flights it had zero problems, zero delays.
We were going on the day that was predicted on the time.
Countdown to six seconds.
You can feel the engines.
Everything starts to rumble.
The engines start up.
There is what we call a steam gage, a vertical bar which shows the engine power.
Center and right engines come up to 100%.
The left engine comes up to 40%.
The pilot calls out left engine at 40%.
In times like this, your mind is working pretty fast.
I remember thinking to myself oh, damn, we are going to have a pad shutdown, we have got to go back to Houston and back to the simulators.
I mean stupid things to be thinking about at that time, but that is sort of the way it works.
And all of a sudden kaboom.
I feel this big kick in my back.
The solids have lit.
What in the hell is going on?
We were supposed to have a pad abort.
Now we are going with an engine.
And, actually, when you are going through maximum dynamic pressure, max q, the engines throttle down to about 65%.
And there is a malfunction if one of your engines gets stuck it is called getting stuck in the thrust bucket.
If it doesn't come back to full power you are RTLS abort.
Well, we were taking off with one engine at 40%.
It sort of dawned on me that we were going to be the first crew to do an RTLS, so at that point I start going through my checklists.
And the pilot calls to the ground we have ignition, left engine at 40%.
And so, for about the first 15 seconds or so, I just had barely time to get out the RTLS checklist and start getting ready to read the procedures.
And then it turns out that the ground has more insight than the crew does.
And it turns out that there are several sensors, one of which feeds that gauge.
But, in fact, that sensor had gone bad.
And, in fact, the left engine was performing nominally and the ground was able to confirm that both by the other sensors and by looking at the acceleration.
And so, everything was OK, I put away the checklist and enjoyed the ride.
But, first of all, it was kind of a scary situation.
But it also shows the difficulty.
Now, suppose we, as the crew, had been responsible for declaring an abort.
If you are going to build in a system where this is the crew's responsibility then you better design your instrumentation so that the crew has all the instrumentation required to make that decision correctly.
Similarly, if this were to be an automatic abort, you've got to have failure detection, identification, reconfiguration.
The last thing you want is to go into an abort situation when you don't have to because there is no way that an abort is going to be anywhere near as safe as a nominal mission.
Obviously, it's just a good story now.
But I think it does have something to inform about how you are going to have to design abort systems.
And, as both Aaron Cohen and Chris Kraft said, although the astronauts always felt comfortable with their abort system but I am not sure that all the people in Mission Control were quite so comfortable knowing the things that might go wrong with it.
And, in fact, as he said, they always breathe a sigh of relief after first stage when the abort rocket would detach and fire itself away.
Yeah.
If one of the engines is not working or not working properly, I guess the other two engines try to do the work [NOISE OBSCURES].
Well, they certainly will throttle up to 109% if they need to, but they cannot make up for the first engine when you're in the first stage and you have lots of gravity losses.
In fact, when you get, like I said, to about 3.5, 4 minutes then you have enough altitude and downrange velocity to make it across.
And then at some point you get to the situation where you basically gained your altitude.
And, although you are not at orbital velocity yet so you still have some gravity losses, the gravity losses are small enough that if you have a loss of an engine you can still make it to a lower orbit.
That is called abort to orbit, ATO.
And you will hear the call press to ATO means that you can now make it to a lower orbit which is the safest thing to do with two engines.
And then a little bit later on you will hear like single engine TAL which means if you lose two engines with only one engine you can make it across the Atlantic.
And then later on you will hear single engine ATO and then finally single engine.
Then you will also hear press to MECO which means at this point if you lose an engine you can make it through your nominal orbit.
And usually about the last call a single engine press to MECO which means even if you lose two engines you can still make it to MECO.
What happens then if there is an instrumentation problem [NOISE OBSCURES]?
The crew will never declare an abort on their own because it is just realized that the ground has so much more information.
The only exception is if you lose comm.
If we had lost comm.
and we saw our engine at 40%, I mean there were a few other displays which the pilot was starting to call up.
And it could be that he would have figured it out perhaps, perhaps not, but that is the only circumstance under which the crew would make that call.
There was abort at some stage.
What happened to that?
Did they manage to make it [OVERLAPPING VOICES]?
I was trying to remember.
Did I talk about that in class?
Well, all right, I will run through that because it was, again, a very interesting story.
The things that you are afraid about is that if things go back in a hurry in an engine that an engine can actually blow up.
And it is one thing if an engine gets shutdown.
Now you are just flying on two engines.
But if an engine blows up and takes out your whole rear section you have had a bad day.
The instrumentation is biased towards an early shutdown.
If you sense that something has gone wrong shut it down.
We have got intact aborts.
Better to err on the side of safety, even if that might potentially put you into an abort situation.
And I am trying to exactly reconstruct it.
The thing is that if you lose one engine now you are abort ATO.
Now, if you lose a second engine you might be in a situation where certainly you cannot make it to orbit.
And, because you have changed your trajectory, you might not be able to make it to a good Trans-Atlantic landing site.
So, at that point, if you have lost one engine there is a switch on the center console where you can override the engine shutdown command.
What you are now saying is we are in an unsurvivable situation if we only have one engine.
And, therefore, we will take the extra chance of getting an instrumentation caused engine shutdown.
We are not going to take that chance so we are going to disable the ability of instrumentation to shut down the engine and we will just take our chances.
The ground will continue the calculation.
And, as soon as you get to the point where you have the capability of doing a single engine TAL or a single engine abort to orbit, you re-enable the instrumentation.
What happened is the Shuttle launched.
It must have been about 4 or 5 minutes into launch because they lost an engine, it shut down automatically, they are now abort to orbit lower orbit, but they were abort to orbit.
Take the switch, put it to inhibit.
Now, the main propulsion flight controller was very good.
She realized right away that it was an instrumentation problem because sometimes you can see certain trends or you see things change in a way that tells you that this is not the way a real engine behaves, that this is more typical of instrumentation.
She suspected she had an instrumentation problem.
Now they get a little further along in the launch where they could have potentially done -- They were too far to land in the normal TAL sites which were in the daytime on the West Coast of Africa, but they could have made a nighttime landing at some emergency airport in the Congo, I think it was, if they had lost another engine.
But, by the flight rules, they take the switch back to enable so that now, because you can "safely" lose a second engine you want to protect yourself against an explosive situation.
So, now they are enabled.
Now the flight controller sees that the instrumentation on one of the other two engines is starting to go back the way the first one was.
And she realized that in about 15 seconds that engine was going to shut down so she immediately called the flight director and said flight, take the switch back to inhibit.
And this is where the discipline becomes very important, luckily flight didn't get into a long involved discussion about why do you want to do that, what is going on, but just said CAPCOM engine switch to inhibit.
They took it.
And sure enough the instrumentation went bad but the engine was OK.
They got to orbit.
Then they used some of their OMS propellant to raise their orbit a little bit.
That was a Spacelab mission.
And they had a good mission.
But the flight controller actually won a big award for that because she really saved the day.
So, yeah, that is the closest we ever came to a real intact abort.
Yeah.
Two questions.
The first one is going back to the return to launch site.
Do all of the main engine propellant get used up before the tank gets dropped or do you not burn some of the propellant?
You know, I don't know the answer to that.
I don't.
And the other one is on the pad, with your flight, wouldn't the sequencing computer shut down the other engines if it detected that one of them had failed?
And so it must have used the good sensor.
Yeah, that is right.
And, luckily, our little gauge was just fed by one.
And, in retrospect, you can say probably that's not a good way to design it but that is the way it was designed.
You're right.
If the engine controller senses with a majority or however they program it, I don't know the details of the guts of the software there.
But if it is convinced that one of the engines is not working right it shuts down all three engines, which is what happened on four occasions.
Yeah.
When you talked about the forced pitch over in the return to launch site, was that just the gambling of the main engines that gives you that?
Yeah, because you are off the solids at that point.
And that is the thing.
I mean with the solids there is such an enormous thrust and the aerodynamic loads are huge.
You don't want to deviate from your planned trajectory because one of the things that they do is program in load relief for the aerodynamic surfaces.
They take the day of winds launch.
And, if you have wind shears or other atmospheric high jet streams that you are going to fly through, they actually program the elevons and the body flap to move in such a way as to relieve the aerodynamic loading on the wings.
And that is preprogrammed in.
It is not a closed loop.
It is totally open loop so you don't want to change the trajectory once you've put all that load relief in.
How long does that take?
It's pretty quick.
It's about ten seconds, if I remember from the simulator.
It is a sporty maneuver, I will tell you.
No RCS that goes into that?
Oh, the RCS is trivial compared to what you're getting out.
You've got 500,000 pounds coming out of each engine.
The primary RCS is 750 pounds.
But, like I said, they do then open up the RCS as you are flying back.
And what they do is they open up all the engines so that you have symmetrical thrusts so that you are firing out of both sides.
The idea is just to deplete the tanks as much as possible.
The second technical area that I had been hoping that Al Louviere would get into and didn't have a chance to was the payload bay doors which are a fascinating mechanism.
And it is also going to be a segue into EVA because it was a strange situation.
It is kind of relate back to that now when you look at how many successful EVAs have been done and the Hubble repair and the building of Space Station and all the other things, but management at Johnson Space Center was not particularly friendly to EVA back in the `70s.
I asked Chris Kraft about that.
At first he denied it.
He said well, I always liked EVA.
He said well, maybe it was the management of the Astronaut Office that didn't like EVA.
He did admit that Bob Gilruth who was the Director of Johnson Space Center at the time, this was when Chris Kraft was head of flight operations, definitely did not like EVA.
He was afraid of it.
He didn't think it was safe, despite the fact that back in 1973 EVA had saved the entire Skylab project.
Hopefully most of you know that story.
I know it is ancient history.
And clearly we had done a lot of safe EVAs on the surface of the Moon, but basically EVA was looked at it was expensive, it was potentially hazardous.
Given the things that the Shuttle was supposed to do in the original planning, mainly launch satellites, launch pieces of the Space Station, that went away, Launch Defense Department satellites, commercial satellites, EVA was not really part of the big picture in planning.
And, although they did design an airlock for the Shuttle, there were real questions of how much preparation do we have to do for EVA?
Do we really even need it?
Maybe we could save some weight by not having space suits aboard.
That is where we finally ended up.
That is the Hubble Telescope.
This is the side of the payload bay.
I mean, obviously, EVA became a very successful activity.
These are the payload bay doors.
This is in the orbiter processing facility.
And one of the things, well, I have a picture of closing the door.
But the mechanism of these doors is extraordinary.
There are two motors, one at the front and one at the back of each.
Each of the motors has two drive units and they work through a differential so that if one of the motors fails the other one can still drive although at half the speed, just similar to the way a car works.
There is a long torsion rod which runs the length of the Shuttle.
And then that torsion rod is attached to these bars over here.
And I am sorry the picture was scanned in and is not terribly sharp.
As the torsion bar twists then these rods lift up the payload bay door and bring it to a close.
The mechanism, this is the best picture I could find, you notice there is a strong back because, as was mentioned, the doors cannot support their own weight in 1G.
But the system of latches here, you have to remember that the payload bay can expand and contract by several inches due to thermal environment.
And so you have to design a latch system that can accommodate this thermal behavior.
It was an incredible mechanical challenge.
And the complexity of this system, I mean I am always amazed when I look at how the thing works.
I have a schematic of them later, which you can look at, but basically you close the doors.
And then the first thing you do is you want to close the latches on either end.
And then there is also center line latches.
And I think Al Louviere did discuss the fact that these payload bay doors are part of the structural element of the Shuttle during reentry.
You cannot reenter if the doors are not just closed by latched.
You need them latched for structural strength.
There is a series of bollards here, little protrusions which these latches grab on.
And they have designed the rigging of the rods such that the latches that are close to the hinge line actually close before the latches that are further away.
It is a sequential closing of the latches all with a single mechanism so that basically you pull the part of the door that is closest to the torque rod, you latch that down first, and then it is kind of a zipper effect where then you pull it down so that even if the door is a little bit warped thermally you will get the door to close properly.
You wouldn't want to close this latch first and then have the door in a situation where it was buckled out.
And then, of course, the center line latch, because of the thermal expansion, you can have the doors coming together like that or like that.
And you have got to have centerline latches that can accommodate for that.
It is really quite an incredible design.
And Al Louviere had said that he was going to talk about it, but he didn't have time to.
There is plenty of redundancy in here because there are these double motors, but because this is so critical people started to look at suppose you have some degree stuck in the mechanism, it doesn't matter how many motors, you cannot get it closed.
Suppose the motors jam on one side.
Now the other motor is not going to be able to drive it.
So, various things were done to allow for EVA intervention.
And, in the early Shuttle flights, this was the only thing that the crew trained for with EVA, it was just payload bay door contingencies.
For instance, the PDU, the drive unit, this is the payload bay door drive unit or PLBDU.
That is what we call a nested acronym.
That is part of the game there.
You have to go out and actually peel away the liners of the payload bay.
And inside here there is a little mechanism where you can insert a special tool and twist it.
And that actually disconnects that motor drive unit from the torque so that it can turn freely.
And I hope, when you look at these things, you will get some sense of the complexity of these mechanisms.
And so what we do for training -- Of course they build mockups of this in the water and we also look at the real Shuttle down in Florida to actually see these units.
And, for the people who are on the EVA crew, you spend a lot of time crawling around the payload bay getting intimately familiar with a lot of the actual mechanisms just in case you actually have to go out and do something with it.
Then suppose the problem is not with the drive unit but that one of these latches get jammed up.
This is actually a tube cutter.
It is a little hard to see.
I don't know if you are familiar with the things.
They are on a ratchet.
And, as it gradually cuts, you push the blade in bit by bit.
And then eventually you cut away.
And this gives you an idea that they just gave us a lot of tools, a pin extractor, a crowbar, vice grips, you name it.
How much did that crowbar cost?
Well, the crowbar probably cost about $10 at Sears.
And then they had to put on all of the Velcro and the tethers to go in the tool thing, and that probably cost a few hundred dollars.
And then they had to make a drawing of it, and you know how it goes.
EVA is very expensive because everything has to be custom made.
I mean that is one of the things that, because of the constraints of the suits and the gloves, you cannot, in most case, just use regular tools.
And, as I say, everything is tethered.
And I will show you pictures later.
We have a little mini workstation.
They built little receptacles on the side of the suit because it is a hard upper torso which can take a certain amount of load.
And so you plug basically a tool caddy into your suit.
And then you hang all of these tools on the tool caddy.
And you will see some pictures later of how that works.
If the motors don't work and you have now freed the doors so that the door can move but you have no motor to drive it, now we have a mechanical winch and a rope on it.
And I'm not making this up.
You actually run the rope up.
These are the little bollards that the latches catch onto.
You run the rope up over that and then you have to crawl out on the payload bay door, which would be a lot of fun, and attach it here.
And then you actually wind it closed.
And we do this in the water.
I mean this is all fully mocked up.
Yeah.
What do you do if you have trouble opening the doors just after launch?
Well, if you cannot open the doors then you come home.
You wouldn't want to.
I mean if there was something seriously wrong with the doors you better come home.
Yeah, we can do this to get them closed.
But you don't want to purposely put yourself in that situation if you know you have a problem beforehand.
Yeah.
[NOISE OBSCURES] We think it could be done with one person.
Normally, we only go out with two.
But in the orbital flight test, the first four flights only had two crew members.
Was of them was EVA trained.
And then what happens if you get the doors closed by the latches don't latch?
They actually made latches that we could put on by hand.
These are the latches for the side.
And this is the schematic that sort of show the latches going on the orders in that order one, two, three and four.
And, again, the way they designed this so that they actually close in sequence but you only have one drive unit is really just a beautiful mechanical system.
But if they don't close then you take this, and I won't go into the details, but you have to spring it around at least two of those.
And that will give you the structural strength to come home.
And then if the center line latches don't close, again, you can look at the mechanism here.
And they have yet a different tool.
Now, this is actually shown on its side, but this is actually on the top.
And this is a bitch in the water because, yeah, your suit is weightless but the tool is not.
And so you are holding this tool.
When you're holding the tool you want to sink to the bottom, and so you are trying to hold on with one hand.
And you have a tether so you try to tie yourself to the top there so that you're kind of hanging on the tether.
But then, depending on how your weigh out is, if you move this big tool back and forth your body is sort of rocking back and forth.
And you are working above your head.
I mean everybody has to do it.
If you are going to be an EVA crew member and you cannot do this job you are disqualified.
But nobody likes it.
It is a real pain.
That is basically how EVA got sold to the Shuttle System.
I will show you a few pictures of general EVA activities to give you an idea of how the system works.
And then I want to go into the question of the interaction between EVA and the pressurization and the environmental control system of the rest of the Shuttle because, again, this is a systems engineering problem.
And you cannot, in most cases, do one thing without it having an impact on something else.
I talked about doing all this training.
Now we are stepping ten years into the future so that is training for the Hubble Telescope.
And, actually, the telescope is so tall that we couldn't get the whole thing in.
The pool wasn't deep enough so we had to actually cut it in half, and we would work on one half and then work on the other half.
Here is an example.
This is me installing the Wide Field/Planetary Camera.
Now, the training, people normally see the water tank.
You're not weightless.
You all understand that.
If you go upside down the blood rushes to your head.
If you're holding onto tools, the tools will fall down.
And of course you don't have these guys when you're out in space.
Angels if you saw them out in space.
If you're moving around large objects, they have resistance from the water, so you need to get a sense of how easy it is to push things around so you don't get things moving too fast.
We also train on air bearing floors.
This is a mass model of the Wiff-Pick.
The same mass, moments of inertia.
And I am up here on a weight relief system.
And you can really get a sense of what it feels like.
And so you are trying to build a kind of muscle memory so that when you get in space you are not trying to push things as hard as you push them in the water.
And you also remember that if you want to stop something you also have to be able to exert a force on it.
Whereas, in the water, all you have to do is stop pushing.
Now we go into space and we are ready for the EVA.
I will sort of take you through what is involved to give you a sense of the complexity of this system.
First of all are tools.
That is what I looked like before all the cosmic rays made my hair fall out.
It is just one of the hazards of spaceflight.
Here you see the kind of tool caddies.
This is for a ratchet wrench.
And we had about 250 different tools.
About a hundred planned to use and the rest were for contingencies.
And we had to take them all out.
We call this our fish stringer.
Sort of like a Portuguese fishermen.
The fisher wives hang all the fish on the string and then hang them out.
And then we take this out on the fish stringer.
This is the airlock at launch.
And it is totally packed.
And, in fact, we had four spacesuits rather than the normal two so there wasn't a whole lot of room.
And the first thing is you go and you start checking the various systems.
And then you have to unpack everything and move it into the flight deck.
That is Story.
We went out together.
This is the hard upper torso.
This is looking down into the inside of the spacesuit.
The back of the spacesuit there is battery and lithium hydroxide CO2 scrubber.
The CO2 scrubber has to be replaced after every EVA.
It is good for about 12 hours.
And normally if you're only going out once you don't worry about recharging the batteries, but since we were doing successive every day EVAs we would have to take the batteries out every night and charge them.
Take the ones that had been charged and put them into the suits that are going to go out the next day.
And just keeping track of which cartridges have been used and which batteries have been charged and how much, I mean there is a tremendous amount of overhead effort just in managing all of this equipment.
And we had practiced doing this a lot on the ground, as you can imagine, before we went up.
The morning of the EVA.
You get up early and eat as big a breakfast as you can because you are not going to get much to eat for the next eight hours.
These are radiation monitors.
For Hubble we were actually up at about 600 kilometers, which is as high as the Shuttle ever goes, so we got really the highest radiation dose that you can get on the Shuttle on a normal mission.
It was about between one and two REMs which is no big health problem.
But typically the dose on a Shuttle flight at a more typical 300 kilometers is more like tens or maybe a little over 100 milliREMs.
They did want us to take the radiation monitor.
Now we are in the mid-deck.
And when you see these pictures you get a sense of when you're doing an EVA the entire middeck is just full of stuff.
I mean there is so much equipment.
You've got the pants units floating over there, plus the two extra suits and all of the auxiliary equipment.
You try to keep things organized.
Then for thermal control, the original Apollo spacesuits were designed for daytime use on the Moon when it is very hot.
And, in addition, people are walking so they are putting a lot of metabolic heat into the suit.
And so they were very concerned about being able to have sufficient cooling.
They developed a very, very efficient sublimator cooling unit.
You have a little layer of ice that is continually fed from the bottom and it is sublimates.
That basically is how you get rid of your heat.
And then you run your cooling water underneath that ice and it cools off.
And then it is run through what they call LCVG, liquid cooling and ventilation garment which has lots of Tygon tubing that goes through it so that it carries the cold water next to your skin.
And also the oxygen that comes into the suit comes through the helmet over the back of your head so it flows over your face so you breathe the fresh oxygen.
But you would like to get ventilation through the entire suit so you don't build up lots of humidity in your arms and legs.
What you do is the return valve actually comes through these air ducts.
And so the return is picked up at the bottom of your arms and at the bottom of your legs.
That insures that the air does get circulated through your arms and legs.
But when we started doing Shuttle EVAs metabolically you are not nearly as stressed.
Plus, you spend almost half your time in the dark and it gets really, really cold.
And this has been a constant problem.
And here is an interesting systems problem to show how everything relates to one another.
We were very concerned about when you open up the doors of the Hubble Telescope, if direct sunlight comes in, it will cause an out-gassing of the internal material which could pollute the ultraviolet mirrors.
The ultraviolet optics are very, very sensitive to organic contamination, so under no circumstances could we have sunlight penetrating the interior of the telescope.
But we were going to be spending a lot of time with the doors open, so how do you insure that you will never get the sun coming in?
Well, the Hubble Telescope is sitting up here like this.
If that is the sun, just make sure that the belly of the orbiter is always pointed towards the sun.
Then you never have to worry about sun coming into the telescope.
But what you do have to worry about is that, well, during the dayside of your orbit, if the sun is over here and the earth is over here, during the day your payload bay is going to be pointed towards the earth.
And so, you basically have a radiative heat exchange with something that is at about 20 degrees C.
No big deal.
If you go around the other side now, you at nighttime, you're pointed at deep space.
And so you're radiating towards absolute zero or three degrees Kelvin.
And that is a concern.
And you get very cold.
They were predicting temperatures of as much as 150 below zero Celsius.
We were very concerned not only were our hands going to get cold but some of our tools might not work.
We pushed very hard for a test in a thermal vacuum chamber.
Our tools are stored in a toolbox outside in the cargo bay.
And we wanted to go through going out and taking the tools out.
And, of course, they are held in there because they have launch locks so there are little pit pins and latching mechanisms that you have to remove so that you can get the tools out.
I went in to do the first test.
And it is a vacuum chamber.
And the walls are painted black.
And they can run liquid nitrogen through the vacuum chamber.
And they could cool it down to the lowest temperatures that we were expecting to experience.
I opened up the toolbox and about 75% of the tools the launch release mechanisms were just frozen shut.
I couldn't get them out.
So, that started to get people's attention.
They sent the engineers in to redo some of the tolerance.
They played around with the lubrication a little bit.
Then Story went in a week later to do his run and he was able to get most of the tools out.
I think he got all the tools out, actually, because I was only in for a few hours.
Because once we realized that it wasn't working I came out.
He was in there.
I remember he said at one point just holding onto these tools my hands are really, really cold.
I don't know.
About an hour or so later someone asked him how his hands were.
He said oh, they are fine, they've warmed up.
Well, Story is from the South.
Those of you with experience in winter know that if your hands are really cold and after a while you don't feel them anymore, it doesn't necessarily mean that they have warmed up.
When they took him out of the chamber his hands were deep purplish black and he had severe frostbite to the point where they sent him up to Alaska to some frostbite specialists.
In the end, he was very fortunate.
He got off with no major permanent damage and was able to fly.
But, as you can imagine, that got serious management attention.
So, gloves now have electrical heating units in them.
But we didn't have that available.
What we had to do was to change the attitude profile so that we were basically pointing towards the earth.
Now, that is generally OK.
If you are pointing towards the earth and the sun is coming from your belly you are not going to hurt the Shuttle, but a quarter way around the earth you do have the possibility now that the sun is going to hit the telescope.
What they had to do was to do two attitude maneuvers every orbit in order to basically keep us in a more benign thermal attitude for the EVA.
But to prevent the sun from shinning on the telescope.
And we didn't the reaction control system propellant budget to support that.
Remember we were going into a high orbit which meant we needed to use a lot of OMS fuel to get up there?
And we needed a lot of propellant for the deorbit because the higher you are the more you have to burn to get down.
And so the flight control community, together with the pilots got together and developed a special way that we could do maneuvers using only half the number of jets that you normally use.
And so this is an interconnection between the thermal environment of EVA and the reaction control propellant budget which nobody in the early days of flight planning would ever have believed that there is this sort of connection.
But it is just another good example of how these systems all play with one another and you cannot make changes in one without another.
I am going to finish this unit and then we will take a little break and then I will do the last part.
The process of getting in the suit.
First you get in LTA, lower torso assembly, basically the pants.
And, of course, this is one time when you can put your pants on both legs at a time.
You cannot do that too often.
But, again, look at the middeck here.
It is full.
This, by the way, is the escape pole.
We talked about this earlier.
During launch and entry it is actually bolted into position so that it will take you out the door, but once you are in orbit you just sort of float it up and out of the way and it just kind of Velcroed to the ceiling.
And, of course, it has no weight up there so it is perfectly happy.
You get in the pants.
Now, the upper torso of the suit is attached to the wall inside the airlock.
Now you have to float into the airlock.
And that is what you're looking at when you're getting into the suit.
There are a lot of connections you have to make.
This is the water bag.
This is the comm.
connection for your Snoopy cap.
This is the water and air connection which you have to hook into your LCBG.
And these are the arms that you have to get into.
And the problem in the design here is your shoulders are wider than your chest.
When you are actually inside the suit you want the distance between these two arms to be conformal with your armpits.
Because, if it is out here that cuts way down on your mobility.
But if it is too narrow you cannot get into it.
That is the basic design problem in this sort of a suit.
Now, the Russians have a rear entry suit that doesn't have that problem.
It is always a challenge and you really have to push and struggle to get in.
Eventually they wanted to design this suit so that one person could get in by themselves, but it cannot be done.
I mean you can get in like this but you just cannot pull up the waist ring and attach it by yourself.
Nobody has been able to do that.
It is a great feeling of accomplishment when you finally get inside.
Then you put your helmet on.
Now you're starting to see what we are dealing with because we have to take all this stuff outside in addition to the tools that are already outside.
These are the plugs which we put the mini work station.
We haven't put it on quite yet but it is just a whole bunch of equipment which you have to manage.
At this point we purge the suit with an oxygen flow.
Now, remember, we are at an atmospheric pressure, or almost an atmospheric pressure, I will get into that later, of pure oxygen, so that also puts very serious flammability constraints.
Although, when you go outside, you are only at 4 psi.
When you are inside here you are at the full cabin pressure.
Actually, in order to do your leap check you have to go to 4 psi above cabin pressure.
You are actually working at above one atmosphere of pressure of pure oxygen, so that is a very serious flammability design constraint.
And we have to sit and breathe pure oxygen then for about 40 minutes to get the nitrogen out of our blood.
And, again, I will be discussing this whole atmospheric and bends problem.
But, if you don't, when you drop down to 4 psi, the nitrogen in your blood bubbles out and you get the bends, just like a diver who comes up too fast from a dive.
Just one or two pictures of what went on outside.
There is Hubble.
I was changing some fuels there.
It was kind of neat working underneath the solar panel.
Those were the old ones which we took off and replaced the next day.
But you have to be really careful because you don't want to bang against it.
And, of course, you cannot see above your head, so we always have other people looking at us.
This is the kind of situation where if you had a little heads up display where you could get this sort of a view to show what you're really doing it would really improve your situational awareness.
But, at the moment, we don't have that.
It takes not just the people outside but the people inside paying full attention, reading procedures.
Historically, we carried all of our procedures on a cuff checklist, like so.
But the procedures for Hubble and some of the other flights are so complex that you just cannot do it.
So, nowadays, these are just emergency procedures.
I will pass this around.
I would like it back.
Let's see.
After putting in the new Wiff-Pick, ground had to do some tests on it, so I got Claude to fly me out over the arm.
And we had one of the old Lunar Hasselblad cameras which they let us carry.
Normally, we just use Nikons on the Shuttle, but they let us take a Hasselblad.
That gives a view of what the payload bay looks like EVA.
Here is Story over here working on one of the other things.
The Earth is not flat, don't worry about the picture.
I went around it many times.
And, I mean, some parts of EVA, I really should share it because it just gets really spectacular.
This is about 50 feet, I don't know whether to say above or below or whatever of the Shuttle, but you're just out there in the middle of nowhere.
And, at this point, I was the free-floater.
One of the people is always attached to the end of the arm, which is good when you have to move around big pieces of equipment because now you can react the forces with your feet.
When you are a free-floater either you're in a foot restraint, which limits your mobility, or one hand has to actually be holding you so that you can react forces.
Of course, we are attached by a waste tether, which is then attached to a long real of stainless steel.
But you can set it so that the springiness is taken out.
And so I could basically, and I did from time to time, must sort of let go.
And it is a really neat feeling because once you convince yourself that you're not going to fall down, which I'm enough of a physicist to understand the orbital mechanics, but, nevertheless, the first time I let go it was an interesting feeling.
The thing is when you're holding onto something, whether by your feet or your hands, of course the Shuttle is so much more massive that you feel yourself physically related.
You are controlled by the Shuttle.
And so the Shuttle is your point of reference.
Maybe the Shuttle is in orbit but you are attached to the Shuttle.
As soon as I let go, the physics totally changes.
And it was really this transformation into being a human satellite.
I mean I really felt like I was a satellite now.
I wasn't attached to the Shuttle.
And sometimes, if I could turn around before I let go so I didn't see the Shuttle, it was really kind of neat, especially at night, just sort of floating there and all the stars and everything.
Yeah.
Do they build in time for you to be able to kind of do that kind of thing?
No, they don't build in the time.
But, like in this case, two days before on Story's and my last EVA we had replaced -- Not replaced.
These are the magnetometers up at the top.
The gross maneuvering of Hubble is done by reaction wheels, but the reaction wheels, when they get spinning too fast, you have to be able to desaturate them so they have magnetic torquers, these long torque rods which create a magnetic field.
There are no jets on Hubble because it would cause pollution of the optics, so you need magnetometers up at the top to sense the magnetic field in order to operate the torquers.
The magnetometers were never designed to be replaced.
They weren't supposed to fail.
Well, both of them failed in the first couple of years.
We couldn't take them off so they designed two new magnetometers that we could actually insert and bolt on, on top of the old ones.
And then we took the electrical and data connections and hooked them up.
But I noticed on that day -- We were very concerned with quality control.
First of all, that we didn't break anything that wasn't already broken but also to look for any signs of damage because, you know, this was a telescope that was supposed to be maintained.
We saw a little bit of paint chipping off the outside of the old magnetometers.
They were concerned that they might float around and get into the optics.
We went into the payload bay the next day, the other EVA team, and they cut off some of this gold insulation material from one of the thermal enclosures that some of the equipment was in.
And I'm not supposed to stand in front.
I keep forgetting that.
We made those covers, and then we had to go up and install them.
But, actually, after we installed them then the ground wanted photographic documentation.
We took pictures.
And then the crew inside got their telephoto lenses and they took a lot of pictures.
And then they got the television camera on the end of the arm to take pictures.
And so, during all that time, you do have a little bit of free time just to sort of enjoy the environment.
It would be a shame not to because it is such a spectacular place.
Yeah.
How do you work when there is not light when you're on the dark side.
Let me turn the laser pointer on here.
Oh, no, what did I do?
That is a good time for a break, right?
Take a two-minute break.
And then, of course, when the sun rises you know it so you can turn your lights off.
OK.
I talked about the necessity of doing a nitrogen purge and pre-breathing oxygen to get the nitrogen out of your blood.
This turns out to be an interesting systems problem because it doesn't just affect the suit, it affects the spacecraft.
Those of you who were here at the beginning saw I was blowing up a balloon which unfortunately popped.
It was only to make the point that you can look at a spacesuit, the arms and legs, cylindrical.
It is like one of those balloons.
And when you try to bend the balloon it doesn't like to stay bent because you are compressing the gas and you're doing elastic work on the material of the balloon and it wants to snap back.
And spacesuits basically work the same way.
The old-fashioned pressure suits that test pilots used to wear, which were the genesis of the original Mercury suits, they basically stiffen you.
And, in fact, the launch entry suits that we use on the Shuttle are not designed for joint mobility.
They are just pressurized.
And we do a pressure check before the mission when we're getting suited up.
And they blow it up and you just go like that.
You can move a little bit, you know, enough so that if you're sitting in your seat with a seatbelt holding you in form so that your waist is bent, you can move your arms enough to get to the controls.
But I certainly wouldn't want to try and go out and do any useful work in that.
It has really been an extraordinary design process that people have been able to develop spacesuits with articulating joints.
I mean it is certainly not like walking around and doing things just with your body, but it is amazing how flexible spacesuits are.
And it is a continual challenge to develop, particularly for the gloves.
And, when we get back to the moon, to have legs that can actually walk in so that you don't have to hop around all the time.
Although, there are times when that is good, too.
But, in any case, the stiffness of the suit, because it turns out that most of the stiffness comes from compressing the gas, you want to try, first of all, to build articulated joints that don't change their volume.
And, actually, people have designed metallic suits that look like Robby the Robot.
So, they truly are zero delta volume suits.
The problem is they are very heavy and there are other problems with metallic suits.
And I don't have time to go into that.
Given that you're going to have some volume change, the amount of work that you do is thermodynamically pressure times volume.
You are going to have a delta V.
You want to reduce the pressure.
The lower your suit pressure, the easier it is to bend.
Basically, you don't want to fill your suit with any gas that you don't really need.
We certainly don't need nitrogen to stay alive, not on a short-term basis, so we fill the suit with oxygen.
And we run it at about 4.3 psi for the Shuttle.
This is just historically Mercury, Gemini and Apollo, the suit pressure was a little bit lower.
Doctors felt, after doing calculations, what you're really interested in is the partial pressure of oxygen in your blood.
And they wanted a little bit more margin.
Since the Shuttle was supposed to be operational this and operational that, they wanted a little bit more margin.
And so we bumped up the suit pressure a little bit.
Mercury, Gemini and Apollo cabin was 100% oxygen at the same pressure so that you didn't have to worry about pre-breathing.
In Skylab, it was not pure oxygen but it was a high enough oxygen that, again, they didn't have to worry about pre-breathe.
It wasn't until we went to the Shuttle, which typically the Shuttle is at 14.7 or 100 kilopascals normal atmospheric pressure.
If we know we are going to be doing a lot of EVAs, and I will talk about this later, what we do is we drop the overall cabin pressure, but we keep the partial pressure of oxygen the same so you end up bumping up the oxygen concentration.
We don't do that with the ISS.
The decision was made by the life scientists that since they wanted to study biology on the Space Station and all of our database is at one atmosphere, that if they took the Space Station down to 10.2 psi basically it would invalidate all of their life science research.
So, the Station was only designed for one atmosphere.
The operational people, when they started to talk about how much EVA was going to be involved in building and maintaining the Space Station, the astronauts said this is crazy.
And you will see the impact of this later.
This is just looking in the future.
These are decisions that are going have to be made for future space systems, and they are looking at a lower working pressure.
And you will see why as I go through this presentation.
I think I'm not going to go through those charts.
Again, this is a system.
So, you are affecting a great many different things.
Let's see.
Which is the laser here?
There we go.
One of the interesting things here with EVA, just like in any ECLS system, is that the human body becomes one of your subsystems that you have to take care of.
And, in many cases, we don't have a whole lot of design flexibility with our bodies because they come predesigned.
So, we have to deal with that.
We have all of the physiology, the bends.
Materials. I talked about flammability in a pure oxygen environment.
I talked about the constraints of microgravity and partial gravity physiology studies in the ISS.
Of course, NASA has decided that we don't need to do that anymore.
And, I guess, our international partners are still going to want to do this.
In any case, the Station is designed.
The cooling we talked about, I think, in the ECLA section lecture, the fact that if you drop your cabin pressure now you've got to pump more air for all the air cooled things.
And if you really want a design for a variable cabin pressure then that flows over into the cooling requirements, more water cooling, different fan requirements and so on.
And it goes on.
If you are going to do multiple EVAs, what you're really interested in, when you're looking at efficiency, is what we call EVA Work Efficiency Index.
It is the total amount of time involved in preparing for and carrying out an EVA, the ratio of that to the actual useful working time you get outside.
And the more time you have to spend breathing pure oxygen to denitrogenate your blood, that is taking away from your overall work efficiency.
When we do tests on the ground and we start out at normal sea level pressure and we want to go down to 4.3 psi pure oxygen, we actually have to do a four hour nitrogen purge.
We have to just sit in the suit or stand in the suit for four hours, this is in the EVA test chambers, before we actually can go down to pressure.
If you want to get an eight hour work day of EVA, that is just unacceptable.
And that is why they made the decision to lower the Shuttle's cabin pressure.
Then you only have to do about a 40 minute EVA.
And I have some of the specific numbers on that later.
As I say, just the review here is that we do have this capability on the Shuttle.
We don't have it on the Station.
They have figured out a few ways to make the denitrogenation process a little bit more efficient on the Station, but it is still a big overhead hit.
And we are very concerned for the future because EVA is not just an afterthought when you're going to be exploring on the Moon or Mars.
You are going there basically to do EVA.
Otherwise, why bother?
We have got to deal with this.
Here is the basic physiology that we are dealing with.
Oxygen percentage on the abscissa, total pressure on the ordinate and all different units.
Millimeters of mercury, psi, and I think now people use kilopascals, which I don't really relate to.
But I know about 100 kilopascals is about one atmosphere, so luckily that makes it easy.
Normally, we are at one atmosphere 20% or 21% oxygen, we are up here, and this is the normal sea level equivalent.
You start dropping the pressure and you have to increase the percentage of oxygen.
There is a maximum level of breathing oxygen at pressure oxygen toxicity because oxygen is almost totally absorbed through your alveoli.
And normally our alveoli stay inflated because we have 80% nitrogen which is only very reluctantly absorbed.
I mean it does get through, that is how it gets into our blood and so on, but it is a much slower transport.
But oxygen gets right through.
And if you breathe pure oxygen for a long time you can really hurt your lungs and it can be lethal at a certain point.
We actually don't have any good physiological data.
I was down in Houston last week at a big EVA conference.
That is why we didn't have class on Tuesday.
They were talking about what about long-term planetary EVAs, even at 4 psi pure oxygen, is that going to be harmful to health?
We honestly don't know.
I mean that is an active area which needs research.
On the other side, of course you get into hypoxia which you have all heard of.
You get it when you go up on Mount Everest and so on.
So, you've got a boundary that you have to work in.
Now, this is sort of where things have fallen.
The blue line is the hypoxic boundary.
The green line is normal oxygen.
When we were using pure oxygen spacecraft environments, we were well above that.
You would like to stay as close as possible.
With Shuttle EVA, we moved a little bit closer to hypoxic, but we're still in a physiologically perfectly reasonable environment.
Decompression sickness, just a quick review.
Any scuba divers here?
You are all familiar with this.
There are various levels of decompression sickness anywhere from just a mild tingling of the skin to joint pain to phase three DCS where you get central nervous system impairment which can actually cause death, so you don't want to mess around with it.
People refer to this famous R value.
You run into this all the time.
What you are really interested in is what is the ratio between the actual partial pressure of nitrogen in your blood compared to your suit pressure?
And this over here shows this R value.
If you are down at one the nitrogen isn't going to bubble out at all so you have no incidence of bends.
VGE, venous gas emboli.
And then, as the R value increases, the dotted line gas emboli, that just means you have bubbles forming.
DCS means actual symptoms of bends.
And then grade three DCS which is very serious indeed.
The way they make these measurements, they have these large physiological studies where they actually get people to volunteer to go through these pumped down protocols.
And then they actually put sensitive microphones and ultrasound things that can actually hear the bubbles as they move around your veins.
I have never been able to figure out why anybody would volunteer for these experiments.
I take my hat off to them.
Because the only way you build up this data is that some people actually do get the bends from these experiments.
And, of course, they have hyperbaric chambers which, as soon as there are any symptoms, they put them right into the chamber and pump them to pressure and it goes away.
And I don't think that they have lost any volunteers.
Like I say, that is not something I am going to volunteer for.
Other factors, this is something that becomes important.
It turns out that the amount of time you spend at reduced pressure is important.
Also exercise.
It turns out that the more you exercise, maybe it makes sense, you're moving your joints around, but the bubbles come out.
Actually, what they have started to do on the Space Station, since we cannot go to a reduced cabin, that while they are breathing oxygen for the first hour or so they do very exhaustive exercise, both upper and lower body on an exercise bike with arm exercise as well.
To try to drive away as much of the oxygen early on in the decompression preparation as you can.
OK.
Where does that put us?
As I said, the more nitrogen you have in your blood to start out with, the longer a pre-breathe you are going to have to do before you can go out.
These are the normoxic and hypoxic lines, which I showed you before.
And then these are the contours of the amount of time you have to pre-breathe.
Remember, I told you if you are at one atmosphere, 20% oxygen, your pre-breathe time is 240 minutes, four hours.
This is to get yourself down to an R value of 1.65.
This is not 100% safe because, remember, at 1.65, there still is a 25% incidence of DCS.
Now, the interesting thing, these are the statistics that come from the laboratory trials, we have never had a reported case of bends during EVAs.
And people are not quite sure why.
Some people suspect that maybe even if an astronaut is getting joint pain they are not going to report it because maybe that would prevent you from doing another EVA.
I mean there are enough other pains that you undergo in just using a spacesuit that maybe you don't even notice it.
You feel a problem in your joints or your fingers and you say oh, damn, my gloves don't fit right or something like that.
I have another slide mentioning this later.
There is some suspicion that weightlessness may have an impact, which means that the bends susceptibility on the Moon or on Mars may actually be greater than it is in weightlessness.
We just don't know.
Here is where we have gotten to on the Shuttle EVA.
Again, this is no pre-breathe, this is one hour pre-breathe, so we are at about 40 minutes on the Shuttle.
That is where we are.
Now, this is a design problem.
How are we going to design the CEV?
How are we going to design the equipment, the habitats that we are going to use on the Moon and Mars?
Now we're talking about surface exploration.
And, as I said, there is a lot of uncertainty about the affect of gravity.
But we suspect that it may be more bends inducing than weightlessness.
And so what they are doing for safety and conservatism is instead of using the R value of 1.65, which we use on the Shuttle, they are taking it down to about 1.3, 1.4.
Now, what does that do?
Here is 1.4, 1.3.
Where does that put the Shuttle EVA we now would have to get down to that R value?
We have got a two hour pre-breathe.
To get down to 1.3, we have got a 2.5 hour pre-breathe.
And this is with the normal spacesuit at 4.3 psi.
We are heading an operational problem.
If you want to go out on your geology traverse on the Moon, you have got to depressurize, denitrogenate for 2, 2.5 hours.
I don't think so.
But what are we going to do?
Well, if you increase the suit pressure now the R value, at a given level of nitrogen, goes down.
And so at 6 psi, even for an R of 1.3, you are in the zero pre-breathe range, which would be great.
People have suggested, well, maybe we should build a variable pressure suit so that you could go out at 6 psi, sort of get things set up.
And then all that time counts towards your denitrogenation so maybe then after two hours into your EVA now you can drop your pressure.
And now, if you have any things where you need more dexterity, you will be OK.
But the pressure control system in a spacesuit is a very complex undertaking.
The Russians do have a dual pressure suit.
They never used the lower pressure, to my knowledge.
And it is a big hit to try to design this into the suit.
And, of course, to design a suit to operate at a higher pressure.
The Russians work at about 5 psi, but it means your suit is less flexible, heavier and is just going the opposite direction from the desire to accomplish useful work, which is why you are putting on the spacesuit in the first place.
This is what we are working against.
Again, we have got a systems problem.
It is not just the pressure.
It is the oxygen.
In Skylab, in Apollo, because you were working in a pure oxygen environment, now, of course, we're talking about what is the pressure going to be in the cabin.
You want to keep the cabin at a higher oxygen environment.
Your material selection is highly limited.
As it says, they tended to use a lot of metallic materials.
Well, what do we know about metals?
Particularly aluminum.
First of all, aluminum will burn at 100% oxygen.
But your cabin, even if it is not 100% oxygen, you are going to have a lot of metallic material.
Well, metal, when you're dealing with radiation, you hit metal, aluminum or even higher atomic number metals with primary cosmic rays and you get spallation.
You produce a lot of secondary particles.
And you end up with actually more radiation than you would have gotten if you had just gotten hit by the incident cosmic ray.
What you really prefer is to have low atomic number.
Hydrogen is best.
Water, hydrogen, oxygen and so forth.
If you are forced to increase the metallic content of your spacecraft because of the oxygen flammability problems now you are going in the wrong direction for radiation protection which becomes an issue when you're dealing with long duration stays on the Moon, interplanetary transport and so on.
So, it is all interrelated.
You cannot change one thing without changing the other.
Flammability.
Well, we have been talking about that.
I won't stay on that.
But, again, it is very dependent on oxygen.
Oxygen restricts the use of non-metallic materials.
But nonmetallic materials are what we would like to use from a radiation point of view.
You would like to have a lot of hydrocarbons, you know, polyethylene plastic in your spacecraft because they absorb cosmic rays.
But, in any case, it is pretty well agreed that we never want to go above 30% oxygen.
It just becomes too restrictive on the types of materials we can use.
That is going to be a design constraint.
And, remember, we are aiming towards a systems level design of our long duration space habitats which are EVA compatible.
30% oxygen.
Now we are back to this.
Now we can draw a red line.
And we have got to stay on the left of that line.
We have got to stay above the blue hypoxic line.
Now, also remember that we have got these pre-breathe lines.
Let's say we are going to limit pre-breathe to no more than one hour.
Well, we are cutting down on our design space here.
We have got the oxygen flammability which is cutting off to the right.
We have got the hypoxic limit which is cutting off in this direction.
And we have got the pre-breathe limit which is cutting off there.
We are kind of in a, I won't call it a box, a rather small triangle.
Now, if we go to a 6 psi spacesuit, as I said, we open up the design space a lot.
But at a price of flexibility, maneuverability and the ability to do the things that we want to do in the first place.
Where are we going to go with this?
As I say, it is an active area of research.
I cannot tell you what the answer is going to be but it looks like they are going to probably be going for 8 to 9 psi with an oxygen concentration approaching 30%.
And that the CEV, from what I have been told, is most likely going to be designed with a variable pressure capability because it also has to be able to dock with the Space Station which means it has to be able to take one atmosphere.
And also, because the CEV by itself is not going to have an airlock, if you would have to do an emergency EVA out of the CEV, for whatever reason, you are going to have to depressurize like we did back in the Gemini days, or Apollo.
And, of course, that is going to affect the design of all the other systems on the CEV because they will have to be able to operate from a vacuum all the way up to one atmosphere.
Just in the end, I hope what you have gotten out of this is, again, we are trying to look at things from a systems engineering point of view.
And this is one big system where EVA cannot be considered just on its own.
I gave an example of how it affects the RCS system with Hubble.
Here it affects the environmental control life support.
Flammability, radiation protection, it is all linked together.
This was the recommendation that we came out with, slightly below 9 psi pushing 30%.
But, as I said, there is a lot of research that has to be done to understand the way bends behave at partial gravity.
How we are going to do that?
I have no idea.
As I said, for the International Space Station, we have to be able to go up to regular atmosphere.
We were making this presentation as part of the study we were doing last year on CEV, but it was so relevant to what we did here that I didn't see any point in modifying it for this class.
But we had to make these decisions on the Shuttle based on similar calculations looking at the design space.
The difference was with the Shuttle we were using an R value of 1.6, 1.7.
And so we were able to get down to a 40 minute pre-breathe.
What we do with the Shuttle is about the day before you are going to do your first EVA you actually drop the cabin pressure.
And when we had the lecture on environmental control, remember there is a cabin pressure controller at 14.7 and a pressure controller at 8 psi.
But when they designed the Shuttle, nobody was thinking about EVA or about this pre-breathe time.
And so, there is no 10.2 controller.
We have to do that manually.
You drop it and then you add a little bit of nitrogen or a little bit of oxygen, depending on what the instrumentation tells you you need.
And then there is a periodic maintenance which you have to perform in order to keep the proper gas concentrations.
And then we leave it down there for the duration of all the EVAs.
And then, when the last EVA is finished, then you turn the 14.7 controller back on.
Now, of course, since you have the proper oxygen partial pressure, most of the gas that comes into the cabin is nitrogen.
And, actually, I think we may have mentioned this before, it comes in the bathroom.
And so, for the time when you are re-pressurizing the cabin, the bathroom is off limits.
And I think that is basically it.
The timing has been pretty good.
Questions?
Comments?
Yeah.
Is anybody looking at using a fully one atmospheric spacesuit?
Actually, it turns out there is a principle called holdings principle.
Let me get this up because I have some pictures of what we were doing.
If you change by less than a factor of two you don't get the bends.
This is an empirical finding.
And so, the idea was if you could have a suit that worked at about 8 psi you would have a zero pre-breathe suit.
And I participated in a bunch of tests.
These are some other robotic things.
This was the hard suit designed by Ames.
Because it is a constant volume suit it is not sensitive to the pressure the way that a soft suit is.
And we did a lot of tests, actually.
We always came unstuck on the gloves.
Nobody has been able to design an 8 psi glove that really gives you sufficient mobility.
I mean it is a dream.
People would love to be able to do it.
If we could figure it out that would solve all these problems.
Although, if you are going to Mars maybe you don't want full pressure because if you have one atmosphere compared to 8 or 9 psi your structure has to be that much thicker and heavier.
They went through some of those calculations.
This is now on the hand with the Space Station.
And they figured that for all the meteorite shielding and everything they had to put on the outside of the Space Station that actually the change in thickness that would be involved in changing the pressure from one atmosphere to another really wouldn't make that much of a difference.
All of these things, again, they are interrelated.
But it is a good point.
That is why I showed those designs for 6 psi because people are still thinking suppose we could design a spacesuit to work at a higher pressure.
But right now we don't know how to do that.
I hope everybody has a very happy Thanksgiving.
We will see you a week from today.
And if there are any questions, again, about either your oral presentations or the written presentations, I will be here today.
And then I am gone for the rest of the week, but I will be looking at emails so you can send me emails and we can discuss things.
OK.
Free Downloads
Video
- iTunes U (MP4 - 228MB)
- Internet Archive (MP4 - 369MB)
Subtitle
- English - US (SRT)