Flash and JavaScript are required for this feature.
Download the video from iTunes U or the Internet Archive.
Topics covered: Environmental Control Systems
Instructor: Guest Lecturer - Walt Guy
Subtitles are provided through the generous support of Heather Wood.
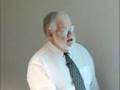
Lecture 13: Environmental C...
Walt Guy's biography is on the website.
I hope you've had a chance to look at it.
I will just say briefly that I guess, of all the lecturers coming, that Walt is the only one who is still actually working actively for NASA.
He is head of the Automation, Robotics and Simulation Division, division chief at the Johnson Space Center.
But that is not what he's talking about there.
He's talking about the job that he used to do for about 20 years which was head of environmental thermal life support and crew systems.
And, of course, the crew system includes all the space suits and the good stuff that I was fortunate enough to get to use.
So I've got a personal debt of thanks to Walt.
Aaron, I think you wanted to say a couple of things so I will pass the microphone to you.
Yes, I would like to say a few words about Walt.
We've worked together for many years.
And Walt Guy has the very unique capability of being not only an outstanding engineer, a technical person, but he also has a very unique capability of being a great or outstanding project manager, program manager.
No matter what task you gave Walt, he could deliver the product on performance, on schedule and on time.
He has that unique capability.
And he's done that from the Apollo Program through the Shuttle Program.
And I think you're really in for a treat to hear Walt talk about the environmental control systems.
He has a lot of information to offer you so, without further ado, let me turn it over to Walt.
Thank you, Aaron.
As Aaron said, we worked together over several programs over a long period of time.
I was noticing the picture.
I'm sure that wasn't put there for me.
But my current responsibilities are the robotic arm you see there.
That is a shuttle arm.
Of course there are station arms also.
Grapple fixture over here on the left.
And the little jet pack on the tumbling crewman there is part of my current responsibilities.
The 20 years that Jeff mentioned, I was responsible for the development of the Shuttle spacesuit.
So it was like it was meant for me anyway.
What I'd like to do is talk about the design.
I know in the syllabus it said environmental control systems.
So where did all the rest of the stuff come from?
If you go back in perspective, the Mercury, Gemini, Apollo Program had the classical thing that aerospace calls environmental control systems.
It was basically a cabin system.
It took care of the living quarters for the crew.
But in the Shuttle era the responsibility of that system expanded to include more than just the cabin and, thus, the very long hard to say name.
These are the subsystem elements of the environmental thermal control and life support system.
The first area I am going to -- These are the areas, the subsystems.
The first area is the cabin atmospheric revitalization system.
This is a long way of saying to take care of the atmosphere in the cabin.
Humans are pollutants and this is the system that takes care of that.
The cabin atmospheric pressure and composition, this is obviously to maintain the pressure and enough oxygen for breathing.
The third is the cabin thermal control, and that is the classical environmental control system.
But, for Shuttle, we've added some functions.
One is the water and waste management subsystem.
There are some hygiene functions required, there are some recharge of the spacesuit's backpack, management of the fuel cell water system, the fuel cells of the electrical power supply and provide a lot of extra water.
Also, the vehicle at this point, has a dedicated active thermal control system that takes care of all of the systems in the vehicle, not just the cabin systems.
And then last is the airlock support subsystem.
This is the EVA crewman's portal to space.
And so the environmental thermal control and life support system covers all these areas.
What I will do is go through each one of those.
The first is the cabin atmospheric revitalization system.
Its functions are CO2 and trace gas removal, humidity control, environmental cooling and atmospheric circulation and ventilation.
Again, I will talk each about these.
But I do want to mention that there is a uniqueness about space and that there is no convection so circulation is important.
Also, because of the fact that there is no convection, getting rid of the body heat, the latent heat requires a ventilation of the crew.
So each of these areas ended up as part of the system design.
This is an overview silhouette of the Orbiter.
I will use this throughout the presentation.
As you can see, part of the system we're talking about now, the atmospheric revitalization system is located upfront where the crew is.
It is beneath the floor.
And we will be talking about the systems in the remainder of the Orbiter as we go along.
This is a simplified schematic.
I would like to start at the cabin fans.
These are redundant.
Each fan will do the job.
The next in line is the CO2 scrubber followed by the cooler, the cabin heat exchanger.
There is a bypass around that for control of the amount of cooling that is needed.
As you are aware, the Shuttle can fly with few or many crewmen.
It turns out, as time has gone on, we fly pretty much a full compliment.
But the original design was to fly a small number of crewmen.
Also, this does give you temperature control.
The same heat exchanger provides the condensation of the water from the atmosphere.
Therefore, you get humidity control.
And then that is collected.
The gas continues on.
It is now chilled and is provided out to the flight deck and the middeck to the crew.
Then the gas from the cabin is then drawn back in through some flight deck avionics and back through the fans.
So this is the basic systems schematic.
I might pause here a moment to sort of explain the role of the government in the development of the Shuttle.
As you know, the prime was Rockwell.
It was their job to build the vehicle.
But the government philosophy, management philosophy was to have ownership of the technical aspects of the design.
So we had basically no excuse.
If it didn't work it wasn't because the contractor did something wrong.
We had to have ownership.
In fact, Aaron, as the project manager, would probably look to us first as the reason for a problem as opposed to Rockwell because we were the conscience of the program so we should have made sure they didn't do it wrong and provided whatever technical insight to Aaron to make sure he made the programmatic decisions to make sure things were not done wrong.
Very early in the Shuttle Program, I was responsible for an engineering organization.
And that engineering organization wanted to own the design of the environmental thermal control and life support system.
We acquired a chamber.
This is a near atmospheric chamber.
It is a square so it is obviously not shaped like the Orbiter cabin, but the volume was appropriate.
And it did allow a sealed environment for testing.
It would take a couple of PSI delta Ps.
That's about all it would take.
We developed a -- [COMMENT FROM HOFFMAN], No, that was a different one.
OK.
We developed an environmental control system or an atmospheric revitalization control system and placed it inside to do early testing.
As you can see here, this is the fan package.
The CO2 scrubbers are here.
Heat exchange package over here.
And you can tell we're not current in terms of calendar-wise.
The CO2 and trace gas removal, the requirements were to maintain less than 7.6 millimeters of mercury.
This is certainly to provide a habitable environment for the crew.
This is higher than sea level but the medics agreed that that was a completely adequate level for CO2.
The CO2 absorption came from the humidified cabin gas so the system had to accommodate that as a feed stream.
The absorbent selected was lithium hydroxide.
The equation was a lithium hydroxide reacted with the CO2 to produce lithium carbonate, water and heat.
And this was a single use system.
It turns out that the lithium hydroxide was an old solution.
The previous spacecraft had used the same solution, but pretty much their solution was unique purchased production of lithium hydroxide.
When we entered the Shuttle era, we were hoping for a long duration.
And the idea was to use the commercial products to get away from special production.
And so we looked around.
And the Navy was producing a screened special production run for their submarines.
So there was a lot of use there.
And so the production was reasonably upo.
We decided to use the Navy grade.
And so we brought it in and put it in the test bed that I just showed you and did some tests, and it didn't work worth a darn.
You notice the loops here show that our use of the canister was much more rapid that we had hoped for.
The nice beautiful deep loops here are a process that we developed to screen the Navy LiOH so that we didn't have to create a new production capability but were able to test the LiOH that came off their production line and get a LiOH that was much more humidity tolerant.
The key here turned out to be not the chemical itself but its ability to produce good performance over a wide humidity range.
So we were able to screen that and make that a viable non-special production run LiOH.
The lithium hydroxide, I said, was an old solution.
All previous spacecraft have been pretty short mission.
And even the Shuttle was a short mission.
But the specification for Shuttle said that it should have a capability of going 28 days, and 28 days is not a short mission, at least not in Shuttle parlance.
The engineering part of the organization said, well, why don't we go with a regenerative system?
SkyLab had just been successfully flown with a regenerative system, something that wouldn't have all this expendables because 28 days worth of lithium hydroxide for seven crewmen fills everything up with lithium hydroxide.
There is no room for anything else.
So we began to look at a regenerative system that could be used.
Actually, it was proposed as part of the original Shuttle development.
But, at that point, the 28-day mission was considered a special case and the design case was the shorter missions.
And, in fact, there were even projected to be a lot of short missions.
The idea that the Shuttle was a space truck.
It would take stuff up, dump it out and come home.
And so the lithium hydroxide was a lighter system than a regenerative system.
The lithium hydroxide was placed into the vehicle as a basic design.
But, when the Space Lab era came along, we needed longer missions.
We flew about four or five times in this 13 to 16 day range, and those missions did require a lot of lithium hydroxide.
And so it was proposed to use an absorbent that was regenerable, and the one that was selected was a solid amine.
The absorbent is a polymerized ethyleneimine.
Its designation is RNH, and I don't really know why.
The absorption actually comes in, in two steps.
First there is a reaction with water to create the Freon.
And then that is then reactive with the CO2 to give you another free ion, but the CO2 is now captured.
The desorption then with the heat and vacuum gives you back your original amine.
So if you pack this in a bed, and what we used was an expanded metal foam heat exchanger that you could fill all the holes with the solid amine and put the passages adjacent to each other so that you could use the heat of absorption to do your desorption on your other side.
And all you would need to do is expose it to vacuum.
It was a very simple system.
It worked fine for the three or four missions.
I'm not sure how many missions it was used.
But it was an adjunct.
It was added in, put onto the floor, and then it was taken back out when it was no longer needed because it was just wasted load.
Yes.
For the lithium hydroxide, the product LiCO3, is that a solid form or a gas form?
No, it's a solid lithium carbonate.
And that goes in the canisters that you remove?
Right.
When you remove the canister it's a big lump.
OK.
Finishing up the CO2 and trace removal, we go to the trace removal and use the simple system of activated charcoal.
And, in fact, it is packed into the same canister that the lithium hydroxide is packed into.
It is a single use.
There is a configuration, I believe, now operationally where the entire canister is activated charcoal so it can be used for cabin gas cleanup.
So, if you had an issue that you needed to clean the cabin up, you could do that with a canister just of charcoal.
The next area is the environmental cooling and humidity control.
I mentioned on the schematic that we have a cabin heat exchanger.
The front part of the heat exchanger basically is your reduced cabin gas temperature.
The backend of the heat exchanger is where the condensing occurs because we get the gas below the dew point and, therefore, the water drops out.
The heat exchanger has the characteristic that, as the water collects at the outlet of the heat exchanger, there were some holes drilled, actually, in the thin passages so the water can be sucked out.
And it has the nickname "slurper." So it slurps the water out.
It takes about 2% of the flow.
So, as the cabin fan produces the flow through the heat exchanger, about 2% is siphoned off in the slurper.
And that carries the water to the centripetal water gas separator.
This is a device that basically spins the airstream.
It's an airstream with water and trained.
It spins the airstream.
The water goes to the outside.
It is collected in a trough and there is a pitot tube in the trough that the water impinges on, and we get about a 40 PSI delta head with that.
It's just a pump basically, a pitot pump.
The atmospheric circulation and ventilation is the next part of the system.
As you know, we're still in atmospheric revitalization.
There are the redundant fans that I mentioned.
Each fan will do the job.
There is also flight deck and mid deck duct system.
It looks very complicated but there are only several points I want to make here.
This is the atmospheric revitalization system I mentioned before.
As you see, it is below the floor.
This is the mid deck here.
This is the flight deck.
So it's below the floor of the mid deck.
The gas exits the heat exchanger.
It goes up.
There is some aft mid deck ventilation exhaust.
It goes on up to the flight deck and exhausts on the flight deck.
Also there is a section that goes on the forward side of the cabin through the forward control console and blows in the face of the crew.
The return is back through some avionic areas to provide cooling, and then back down to the system.
This is a complicated picture, but really it is a very simple flow system.
This becomes important later in our discussion because good mixing turned out to be a really important parameter.
Not just cooling.
OK.
The second.
Remember we had six?
This is the second element.
This is the atmospheric pressure and composition control.
The first job is the 14.7.
The Shuttle selected sea level, the idea being we could not have a lot of special designed equipment.
Shuttle was intended to be a less expensive spacecraft than the previous ones.
And so a sea level atmosphere gave us no questions about human survival and gave us a lot of opportunity for using more standard equipment and not specially designed equipment.
There was a requirement, though, for a PSIA total control, but this was only for an emergency de-orbit.
The problem statement is that you got a hole in your cabin.
Don't know how you got it but you have it.
And the idea is the system has to be able to sustain the crew for a de-orbit.
And I don't know who made up the number but 169 minutes is the number.
We have an emergency return, 169 minutes.
The system has to be able to accommodate that keeping the total pressure at or above 8 PSI.
So there has to be enough flow capability, enough consumables to do that.
The half-inch hole was a programmatic decision.
I cannot speak for where the number exactly came from, but it probably had to do with meteoroid debris kind of analysis that said what type of hole you could expect.
Anyway, that was the design for the system.
The next aspect or element of the system is the O2/N2 partial pressure control.
At the total pressure of 14.7, we wanted sea level which is about 3.2 PSI oxygen.
At the 8 PSI, we wanted it to be survivable but the oxygen could be less.
Obviously, people live in Denver so that works.
There are also crew breathing masks which can be used in conjunction with low oxygen partial pressure.
The cabin had to have both positive and negative relief.
It had to store the oxygen and nitrogen, and it had to provide pressurization for the water management system.
Remember earlier I talked about the more pervasive requirements?
The water and waste management system now is placing requirements on the atmospheric pressure and composition control.
This is a silhouette again where basically here you see the oxygen tank, which is 3300 PSI oxygen tank.
There are nitrogen tanks on both sides.
These are high pressure tanks all 3300 PSI.
The relief valves are shown here.
And there is an O2/N2 supply panel which has all of the valves and regulators and everything on it.
This system, however, though, gets most of its oxygen from the cryogenic tanks.
So that is only just shown as an inlet.
These tanks are basically part of the power control system.
Electrical power is hydrogen and oxygen fuel cell.
We use a lot less oxygen than they do so the storage was integrated.
And then we use the oxygen for most of the uses.
It comes from the main tanks.
This is a schematic.
I will start right there.
This is the cryogenic system.
This is the main cryogenic system.
This is not part of the tankage of the pressure and composition control system.
It goes through a restrictor.
This is stored in a super critical state so the delivery is somewhat limited.
And so this goes through a restrictor so that we don't decrease the pressure too fast in the cryogenic tank.
It comes on down through a regulator that regulates down to 100 PSI.
And that comes on down to two regulators.
One is the normal regulator, the 14.7, and the other is the 8 PSI regulator, both of which are available depending on which cabin mode you're in.
If you go over this way you will see the nitrogen.
The nitrogen goes through its own regulator, and it is regulated at 200 PSI.
And that's important because that is contrasted to the 100 here.
Next the nitrogen goes through an on/off valve, a solenoid that is connected to partial pressure sensors of oxygen.
And, if you're making up cabin gas, you have to make a choice as to whether you want nitrogen or oxygen.
And that choice is made by this system and a simple on/off valve.
There is no sophisticated control system.
This is a simple on/off valve.
If the cabin needs pressure but doesn't need oxygen then this valve is open and the 200 PSI basically backs down the oxygen system.
There is a check valve here, no oxygen flow because you get nitrogen.
At the point that you need oxygen, this valve closes, then there is no 200 PSI anymore.
Therefore, the 100 PSI provides oxygen to the regulators.
It is a very simple design.
Off the top of your head, what kind of failures did you have during qual test that really bothered you?
The regulators were produced by Carlton, and the regulators were derivatives of regulators they had been building for years.
So the regulator functions really were pretty good.
But the early problem we had was the pressure tolerance wandered and didn't have as good a pressure tolerance.
And they did make some mods to correct that.
But the more significant issues got into flow issues.
Their test capability was pretty limited.
And when you're talking about very high flow cases you have to do something with the gas to make the regulator think that it is in an operational environment.
And so we did a lot of testing, and I will show you that in just a second, for the high flow cases.
Let's see.
There is a redundant system.
It is not shown in this schematic but there are two of these, just like this.
This is the pressurization for the water and waste management system you see here.
I would say there is a crossover to the redundant system so that you can do mix and match.
If you have a problem in both systems, you can mix and match the capabilities.
Also, the oxygen system provides the emergency breathing system.
It also provides oxygen to the air lock for recharge of the backpack.
We will talk about that later.
And I believe that's it.
So, for normal pressure, we have 14.7.
And you have the automatic pressure regulator, basically on/off valve and the regulator.
The partial pressure is controlled by the 3.2 PSI which is the solenoid that controls that.
It is a very simple system.
The oxygen partial pressure does have another set point at the PSIA goes down to 2.45 PSI.
And I will show you that in a minute.
For cabin pressure relief, the problem statement really is why do you need relief?
Well, what if one of those lines breaks?
You've got 3300 PSI tankage.
It is all plumed together.
The crew, God forbid, step on a line and break it, and now you've got all this nitrogen rushing in.
You don't want the cabin to explode, right?
So you need a relief valve.
And the relief valve was set at 16.2.
And, again, that is one of the high flow cases that we ended up testing.
The system was designed with three relief valves.
Any tool will do the job.
That is just a redundancy philosophy.
Sometimes you will see two items where either one does the job.
In this case, this is a very critical issue so there is a double redundancy here.
For the negative pressure protection, there is an 8 PSI delta relief valve.
In fact, there are three of those.
You don't need the two to operate, but there are three.
This is for the return that I just showed you.
If you come back with a hole in the cabin, the cabin is at 8 PSI, you cannot reverse flow through that hole.
That is not going to work.
You don't want the cabin imploding on the crew so you've got to make up, as you come in the lower altitude, obviously the higher pressure, you've got to get that gas back in the cabin.
So that's what that is for.
OK.
This is the other pressure vessel that was used as a test site.
This was called the Environmental Test Article.
Rockwell gave it that name.
What they planned to do with this device, when they built it, was to do all the wiring runs and the plumbing runs in there.
It was going to be a mockup.
It turned out they built a rather beefy tank.
In fact, maybe they got one very inexpensively.
I'm not sure how they ended up with it, but they got it.
They did do all the secondary structure, so it had the right shape inside.
And so it turned out they didn't end up using it.
They went onto other methods.
And so it ended up leftover in the program.
We convinced Aaron that he ought to give it to us, and he did.
This is what it looks like in cutaway.
You see here all the secondary structure, it was in boxes but we put it all in.
Foamed it in so that the volume was right, so the cabin volume was essentially perfect.
It had the flight deck.
It had the mid deck.
It had the lower deck for the environmental control system.
But we also asked Aaron to give us the cert hardware, which he did.
So we had the cert hardware underneath the floor.
And the pressure control system we're talking about, actually, is on this back wall.
That is a picture of it.
What we did is faithfully run the lines.
In fact, we even use the same line material, same fittings, same everything.
So we faithfully duplicated inside this test article the volume and the geometry and the system.
All the ventilation ducts you saw, all those were in place.
We will come back to it later, but you'll notice on the back wall of this test facility there is an airlock hatch.
And later on I will tell you where that goes.
But this is the control panel and the nitrogen/oxygen system.
This is test data.
We did some testing to see how the system worked.
This was testing of basically the on/off oxygen control system.
Let me see if I can walk through this simply.
We're working total pressure here, oxygen partial pressure here.
During this first interval the oxygen is on.
What we're doing here is we're breathing it down.
And, when we hit the lower limit so that the regulator wants to do something, then the oxygen partial pressure starts up because the regulator comes on.
Now, the total pressure is maintained because that is what the regulator is supposed to do, maintain total pressure.
But as it maintains the total pressure it is doing it with oxygen and, therefore, the partial pressure is going up.
When it is satisfied, that is enough partial pressure of oxygen, then the solenoid valve opens and you see the nitrogen come on.
Now, you remember the nitrogen feed was at 300 PSI versus 200 PSI.
So there is a little jump.
That is just a mechanical artifact of the regulator.
If you change the inlet pressure it regulates slightly differently because it is a balance system.
You see a little jump there.
And then, during this period, the pressure is maintained.
But we're breathing off the oxygen because we've got the oxygen fenced off now.
And then when the pressure is satisfied and the solenoid goes back the other way then we again start the oxygen again.
So the system worked quite well.
If you notice the tolerance band spec-wise was pretty wide, the system operated on a very narrow brand so we were very pleased with that.
Normally that is important.
Not only because it shows you that you do have a good sensitive system, but you cannot -- Mechanical hardware, electrical hardware, either one never operates down the middle always.
There is drift.
You need a little tolerance in your band here to have a decent system design.
Yes.
Does this change a lot when you have the crew performing high metabolic activity?
The cabin is so large that it really doesn't know.
The cycles would be different, obviously, because you consume more oxygen, you would need more oxygen.
So the on/off cycle would favor longer oxygen cycles.
But, in the big picture, there is really not a lot of change.
What was this time period?
It looks like this was about three hours maybe.
That might go to two hours for a cycle.
I mean not really very much.
Sorry.
Does microgravity have much effect on circulation?
There is no circulation in microgravity.
It just lays there.
You push it or it doesn't go.
Diffusion is a driving force, so if you're really, really patient, even at zero G you can wait, but you cannot stay alive that way.
But, yes, you do have to have ventilation.
Still in the atmospheric pressure and composition control.
This is the emergency breathing equipment.
We used plug-in face masks.
There are some uses for the mask that require a carry on bottle to give portability, but the vehicle does have plug-in capability.
The masks were purge-type masks.
You're probably not familiar much with masks, but there is a mask called a rebreather which basically your lung power does all the work and you only purge enough to keep the CO2 down and you keep rebreathing.
And then the purge-type is every breath is a fresh breath, and you then exhale and the gas goes out in the cabin.
And that's the type that this is because the cabin needs the oxygen.
I mean it's not going anywhere bad.
It is OK that it's not completely spent.
It's good.
That's like a scuba mask, right?
I don't scuba.
Sorry.
But I think the answer is yes.
With scuba, you take in the air and then you blow it all out.
I mean there are scuba rebreathing systems, but the typical scuba gear is not.
Rather than raising your hand, if you make a noise of some kind.
Sorry.
I don't mean to ignore you.
The mask can be used in a contaminated environment and the mask can be used if the concentration of O2 is low to give you a better oxygen level.
This is the PSIA test that we did.
Actually, a series of tests.
But this is data from one.
As you see, we started up in the 14.7 range.
We simulated the hole.
The pressure immediately starts down.
The partial pressure of oxygen we let degrade to about the 2.2 range.
This is a different set point.
Remember I told you on the oxygen sensors we have two set points?
This is the second set point.
And so we have the lower oxygen partial pressure.
The system came down.
The PSIA regulator, which is always online, captured the pressure decay, caught it, and the solenoid valve popped back and forth to keep the oxygen OK because we're losing now.
We're going out this half-inch hole.
And the system worked just fine.
This did have the crew on the masks because that is livable, you can live there, but the mask is a better environment.
This is a mask case.
This was a requirement that came on later.
The design is the design now.
The vehicle exists so we have to now operate the vehicle with accommodations for operationally what we want to do with it.
And a new requirement developed and was imposed on the vehicle.
And that requirement had to do with the space suit for the EVA operation.
The Shuttle spacesuits are basically 100% O2.
And the classical way of going EVA from a sea level environment was a four hour prebreathe.
Actually, as much as six hours depending on how conservative the medics were at the time, that number varied around some, to prevent bends.
But it was determined that if you accommodized at 9 PSI for about 12 hours then you only required a really short prebreathe.
And the suit-up time, which gave you 30 to 40 minutes, actually gave you sort of a free tune-up to your prebreathe as part of your suit-up operation.
And so that was viewed as a much better way to do EVAs than the previous way.
The masks are useful but are not very comfortable.
So, if you have to keep them on for a long period of time, they're not very comfortable at all.
Also the medics were very concerned about mask leakage, that if there was any nitrogen inflow that that could break the prebreathe.
But our system isn't designed for 9.
We have a 14.7 and an eight, but we don't have a 9, so we needed a manual procedure.
But before that could be accepted there were some issues that had to be evaluated.
And we had to do testing on each of these issues.
The first is the flow rate acceptability at 9 PSI.
Obviously, the less dense gas you're not going to get the same mask flow rate.
And we had to make sure that that ventilation was going to be adequate.
And testing proved that it was.
The next is a thermal acceptability.
There are really two aspects to this.
One is the fan itself is cooled by the environment, so if the environment doesn't provide as much cooling to fan itself it could overheat.
Also, the electronic equipment, some of which is air cooled, the less dense gas could be a problem there.
It turned out that could be controlled with load control.
That could off load power and make that acceptable.
The CO2 performance at 9 PSI, we needed to make sure that was OK.
It turned out that, of course, the 9 PSI case, we don't care about the lithium hydroxide.
The purge flow is so great there is no CO2 problem.
But at 9 PSI for 12 hours you have to get performance out of the lithium hydroxide.
So we checked that and that worked out fine.
And the last item was the ventilation adequacy.
We do have a press and depress.
You've got to come down to 9 and you've got to go back.
And both of those adjust the gas mixture.
And so we wanted to prove that that was OK.
And so we went to the facility that we were talking about and ran this profile.
Now, this profile was created by our-- yes?
[AUDIENCE QUESTION] Well, that's what I just said.
Yes, it does.
The air-cooled equipment is going to run hot.
If you want to cool that equipment, it will become a little clearer later, but the equipment is in equipment bays.
And so, with the less dense gas, you need to have some of that equipment turned off so that the cooling that is available is adequate.
So you do load control, get rid of some of the electronics.
And, therefore, the air that's left, the atmosphere that is left can cool the equipment.
And then when do you increase the pressure back to normal after the EVA?
After the 12 hours.
No, actually it's before the EVA.
Well, I'm not sure what they do now.
When you're all finished with your EVAs then you bring the cabin back.
Because, typically, you do multiple EVAs.
And so, from one day to the next, you just keep the cabin at, actually, we use 10.2 now rather than 9, but I think you have to certify it for 9, right?
This was an early certification for 9.
Yes.
But wouldn't it have been easier to simply design the whole system for 9 from the beginning if you had [OVERLAPPING VOICES]?
Well, this was not a Shuttle requirement.
This was an operational requirement that occurred after the Shuttle specifications were set.
You're correct.
If we knew it ahead of time it would have been much easier to do it all for 9 PSIs, exactly.
It would have just been a third condition.
But, as Jeff pointed out, we changed our mind.
The first was 9 and now we're at 10.2, so we're not consistent.
As we learn, we change our mind.
And the 10.2 was selected later.
Our mission operations people that do the crew time lining and all, they created this
And so we tested it. procedure.
This has a simple depress cycle.
You come from 14.7.
You hesitate at about 11 and replenish the oxygen.
You do that twice.
You then do manual control down at the 9 psi level.
You notice the oxygen partial pressure here is always maintained by these adjustments, and also during the manual period you select what you want.
And then when you come back, the procedure was to increase the nitrogen and then finish the top off with oxygen.
That was thought to be completely acceptable because the oxygen level, at this point, is completely viable.
And so doing the nitrogen first and topping it off with oxygen was thought to be an acceptable way to operate.
So we did the test and this is what happened.
We were all nice and comfortable with our oxygen partial pressure.
And you see the area next to where we were repressing.
We depleted the oxygen substantially.
And the mid deck and even the flight deck, we depleted the oxygen.
And I've only shown you half the data.
If you look at the rest of the data, you see where the oxygen went.
These equipment bays that I mentioned earlier, the avionics bays and other equipment bays are basically isolated volumes from the cabin.
So, as the pressure comes up, what you're doing is you're pressurizing these areas with cabin gas, which is oxygen and nitrogen, you're pressurizing these areas with nitrogen only.
Basically, you are depleting the partial pressure here.
You're increasing the partial pressure here.
You remember partial pressure.
You just count the molecules.
That's all you have to do.
Basically, we're putting more oxygen molecules here and we're taking them out of these areas.
And, therefore, they are going down.
This is just a picture of one of the bays.
You see all the empty space around the equipment.
That is where the gas is.
We came up with an alternate procedure which is to simultaneously put the oxygen and nitrogen in together.
And, of course, there is the 9 back to 14.7.
And you notice that solved the problem.
There is still some dip at the supply area because the mixture is lean.
We need from 9 to 14.7, 5.7 PSI total pressure increase.
But we only need 2.5 up to 3.2 oxygen.
This is a mixture, but it's a lean mixture.
So there is still some slight loss, but the only area that came under an issue is near the inlet where the O2/N2 supply panel is.
And, of course, you can just avoid that area.
Well, I should say that that area happens to be right around the bathroom.
And so, before we repressurized the Shuttle, the commander would say anybody who has to use the bathroom use it now because for about ten, fifteen minutes, while we're depressurizing, it's off limits.
OK.
Next item.
This is the water and waste management area.
This is one of the six items.
We have potable and waste water inventory management.
We're continually producing potable water, as you know, and waste water.
We have to dump them, we have to use them, so that is part of the requirement of this
We have to store water for drinking, food prep and waste water storage. system.
It has to be dumped to space.
Commode and urinal for human waste collection.
And we provide water for an evaporative heat sink, which we will talk about later, called the flash evaporator.
Yes.
This is an outstanding example of systems engineers, this total system that Walt is
We can really see the interaction between all the systems and the spacecraft. talking about.
This really is a systems engineering problem.
Potable water, we take all the fuel cell byproduct water and use it.
So it is basically in a fluent to the power system.
We do have a launch storage capability for water and we do provide sterilization.
The sterilization is iodine.
We started out designing a silver ion system.
It turned out that we had manufacturing problems and the company ended up going out of business, so we reverted to iodine.
The Lunar Module used iodine.
Waste water, the condensate from the cabin humidity control and urine and the urine pretreat.
Urine has to be pretreated to bind the urea.
If you don't, you get a lot of ammonia.
It uses a chemical called Oxzone.
It's an acid.
I'm not really sure what it is.
This is where the system is located.
This is one of the simpler schematics.
The water tanks are here.
The water comes from the fuel cell.
As I said, by the way, the delivery is about 150 degrees and 60 psi.
So there is a lot of hydrogen dissolved in the water.
The water is collected in the fuel cells on the hydrogen side, so there is a lot of hydrogen.
There is a hydrogen separator here.
Otherwise, your tanks would end up full of hydrogen which is what you don't want.
You will get water in the tanks.
The hydrogen separator is a silver palladium metal.
It is catalyzed.
It is basically porous to hydrogen but not to water.
If you expose the backside to the vacuum it pulls the hydrogen off and the water goes through just fine.
I will say that there still is a rather high gas content in the water that you drink.
And that gas, of course, evolves once it gets inside your stomach with predictable results.
[LAUGHTER] Yes.
In fact, all sorts of systems that Jeff could explain.
They have slinger systems and all sorts of systems to get separation because it is not really possible to get all the gas out of the water.
OK.
There is a dump system.
There is a potable waste dump to vacuum.
There is also a vacuum vent, which I will show you what that is all about.
And then the flash evaporator that I mentioned, and I will mention more later, is in the back of the vehicle and it has a redundant water feed system both sides.
This is a schematic.
Start at the same place.
Water from the fuel cells goes through the hydrogen separator and then into this bank of tanks.
The tanks are pressurized.
They are yellow bellows tanks.
On the back side of the bellows is nitrogen.
On the forward side, of course, is the potable water.
There is a hydrophobic filter on the downside of each tank.
If a bellows were to leak, you don't want water into your nitrogen system.
So this is a filter to prevent that.
I mentioned the twin flash evaporator feeds.
There they are.
This is the dump nozzle here.
The potable water system provides water to the crew so there is a regular water interface and a chilled water interface here.
I believe that is it.
I remember there were more problems with Apollo than there were with Shuttle.
49:30 I don't have those sorted mentally.
I'm sorry.
I don't remember.
I'm not sure.
[AUDIENCE QUESTION] Maybe that was on Apollo.
[AUDIENCE QUESTION] Basically that means you put your water into a plastic bag.
And now you can actually swing it around like so.
And so the water will go to the outside and the gas is in the inside.
So that actually separates them and you can squeeze the gas out through a valve.
On-orbit trash storage, there is an overboard bleed for odor control.
It's a very small overboard bleed to vacuum.
And the human solid waste collection and storage is also vacuum dried and stabilized.
This is a waste management picture.
The subject we were just talking about is the vacuum vent.
That vacuum vent does vent the commode area where the feces are.
That vent also provides a vent for the water separator.
It also provides a vent for airlock depress for EVA.
So this is the vacuum system.
The urine is collected and trained in a liquid.
Well, let me say that different.
The urine, after it has been separated from the gas stream, is stored in a waste tank, as is the humidity from the cabin atmospheric revitalization system.
And then that is pressurized from the pressured composition control system and is dumped overboard.
This waster is not used for anything.
It is basically dumped just to do inventory management.
The next picture explains a little better about the commode and the urinals themselves.
The urinal originally had a unisex interface cup.
It turned out that didn't work very well, and so later flights there was a custom male/female.
And later there were even some more customizing for the crew, I believe, but I don't have the details on that.
The urinal itself, obviously for urination, there needs to be some way to make the urine go where you want it to go.
And those were done in fan separators.
So there is gas pulled in the cup.
And in training the urine, the urine then comes down and goes through the separators.
This is also a centrifugal-type separator.
And then that is sent to the head that is created here.
Then puts that waste water urine in the waste system.
The EMU spacesuit, it also has a condensate drain after each EVA, and it also goes through this same system and into the waste tank.
The commode.
Basically there is a seat.
The seat has a small opening.
The commode itself is basically a cylinder or a tub.
And there is a slinger in the bottom.
So the slinger basically distributes the paper and the fecal matter on the outside wall in a thin layer.
The vacuum then can dry that so that it deactivates it so it does not end up either any odor or creating any kind of bacteriological issues.
But the entrainment of the fecal material so that it, in fact, hits the slinger, is done with an air flow.
The air flow comes in around the seat itself.
That requires good contact with the seat.
It also requires positioning.
Positioning turned out to be an early issue, or reliable positioning, and so a trainer was put together that had a camera here.
And the trainer, I might add, was discretely located in a non-observable place so that it could be used in privacy, the comings and goings, and also the use of the facility itself.
And they told us it was not hooked up to a tape recorder.
[LAUGHTER] Just to elaborate on that.
Basically, the problem is it really is a rather small opening.
And it just doesn't feel natural to know where to put yourself down.
And it actually did lead to significant messes on orbit.
I won't go into any more detail.
But the idea is that you would position yourself the way you thought it should be, and then you would turn on the lights.
And there is a TV screen in front of you.
I'm not making this up.
[LAUGHTER] So that you could see how close you are to the bull's-eye.
Enough said.
The cabin thermal control is another one of the subsystem elements.
It is a circulating liquid cooling system.
It provides the heat sink for the atmosphere itself.
It also provides the ability to reject that heat to the spacecraft cooling system because you've got to get rid of it out of the cabin.
It has some avionics cold plates, some air cool avionics, support the crew in the airlock and a portable water chiller.
This is the location.
You will notice the avionics bays are listed here with their little circulation systems.
The liquid system then services each of those, the chill or the condensing heat exchanger.
And this is the place that the cabin system connects to the spacecraft system, on the aft bulkhead right here.
We will see the other half of this system in a little bit.
This is a schematic that is very complicated looking but it is fairly simple.
Let me show you the air piece first.
Look at the black lines.
Those are twin fans.
It goes through the air-cooled avionics through the heat exchanger to get rid of the heat.
There are some cold plated avionics in each bay, so you take care of the cold plated and the air-cooled in this bay and in this bay and in this bay.
Now you've got all of the electronics taken care of.
I should have started this up at the pump.
Sorry about that.
Here is the pump and we will come down.
It also takes care of the forward and overhead window mounts around the window for thermal control.
And the hatch is just a way to keep those thermally stabilized.
Back in the early flights, we had something called DFI, development flight instrumentation.
It was a special instrumentation package.
It was also liquid cooled.
And then this is the heat exchanger to the spacecraft system.
It is a Freon system.
This is where the main heat is rejected right here.
On the other side of this you've got cool liquid.
It turns out this is water.
We go through the liquid cooling garment which is the spacesuit cooling garment, the water chiller, go through the cabin heat exchanger to cool the cabin and condense the water out and then back to the pump.
I failed to mention the IMU.
It has triply redundant fans.
It pulls air through it.
And that is also cooled by this gas stream.
Anyway, this is the cabin thermal control system.
These functions, I just said each one of them so I won't repeat them.
Cabins circulating liquid cooling loop.
As I mentioned, water is the coolant.
Water is actually a very good coolant.
And, if you have any requirements that push you that way in your designing thermal control systems, you like water.
Water is completely nontoxic so it can be around the crew.
Leaks don't make any difference.
It has a very high CP.
It's a really good fluid.
The problem with water is freezing.
You don't want to freeze it and you don't want to get it so hot that it turns into steam.
But if you can stay within the boundaries it is a good fluid.
And that is what this is.
Redundant pumps, I showed you that on the schematic.
The liquid gas heat exchanger, I showed you that before.
Cold plates for electronic cooling and the window hatch mount.
The circulating gases that I mentioned for the avionics, the cabin gas was used as a coolant.
You saw the redundant fans and the doubly redundant fans.
I mentioned earlier, and someone commented on it, that the promise early on was the Shuttle could be a less expensive development activity if we used off the shelf avionics, the kind that aircraft use.
And that's a good promise.
It turned out that that's not a very good, not as good a solution for thermal control as liquid cooled.
Liquid cooled is a much more efficient way of providing a heat sink to electrical equipment.
When you blow the cabin gas through it then you end up with the problems of if you change the pressure in the cabin, which we talked about.
That can effect cooling.
Also you have to put out a lot more power.
You have to blow a lot more gas than you do power to move the liquid around, so it's not a very good thermal solution.
And actually the promise of less expensive avionics I don't think was actually fulfilled, although that's somebody else's topic and not mine, because I think everything was pretty well custom.
It did potentially complicate the thermal control system for a good goal.
And how well that goal was met, somebody else will have to speak for it.
Another one of the subsystem elements of the spacecraft.
Well, we usually take a two minute break when we're about halfway through.
Certainly.
Why don't we take our two minutes now and then we will pick it right back up?
[AUDIENCE QUESTION] From an engineering standpoint, it is much better to cool with liquid.
If you use cold plates, it is much more efficient.
You get a good reliable heat sink.
And you can then design your thermal conduction paths so that out of the avionics box you make sure the heat goes to the cold plate.
And you use a lot less power.
You can pump water a lot more efficiently for the cooling capability than you pump gas.
Is that what happens on the Shuttle now or do you have a mixture?
[AUDIENCE QUESTION] This is the air-cooled part of the loop and here are the cold plates.
Let's get the class back.
Thank you very much.
I am sorry.
Did you see what I was pointing at here?
Yeah, you put both.
Yeah, and this is one bay.
And they have both cold plates and the air-cooled here, here and here.
There they are.
Of course, when you go outside the vehicle there is no more air so all the external equipment is cold plated.
Was there another question?
What kind of design changes would you have to make in order to go strictly to completely liquid cooled electronics?
Well, if you're trying to use off-the-shelf and it is air-cooled already, there is a cabinet right there full of it.
If it is air-cooled already then you have to stay with that concept.
If you're custom designing then, from the very beginning, you say I'm custom designing this to be liquid cooled.
And then the internal design is such that there are conduction pads to the base plate so that it can be cooled.
OK.
I guess previously to that all of our spacecraft were liquid cooled.
Apollo was, wasn't it?
Yes.
Because you had to be able to take those spacecraft down the back?
Right.
We evacuated the cabin both in the Command Module and the Lunar Module.
And Gemini, too.
This is the spacecraft active thermal control system.
This is the vehicle level.
So now, even though we're still under this ETCLSS, we are not talking the classical environmental control anymore.
This is a bigger picture.
Also the reason the word active is stuck in here was a left over from previous programs, too.
For example, on Apollo we had several heat rejection systems.
Not a single one.
And we also did a lot of passive thermal control on Apollo.
We had a mission mode called "barbeque" which is basically you roll the vehicle very slowly so you normalize the environment.
If you leave the same side toward the sun all the way to the moon that side gets really hot.
This is the active system to get away from heaters and/or vehicle level control.
There is an on-orbit.
We have a radiative heat sink.
We also have an evaporative heat sink on orbit.
During ascent and entry we have two heat sinks, both of which are evaporative.
We have a circulating liquid system.
We take heat from the cabin, from the fuel cells, hydraulics, all the cold plate avionics and electronics, and payloads, we do cooling for payloads.
All of these are the functions of the active thermal control system.
This, as you notice, is a lot busier than some of the others have been.
You will notice the radiators here on the side wall.
This is the doors.
When closed up there are no radiators.
When opened the underside of the payload doors are your radiators.
And this is on both side, obviously.
That same heat exchanger that I showed you before that interfaces with the cabin is right here.
The cabin load is dumped there.
Fuel cell load is dumped here.
The fluid is a Freon 21.
There is a Freon 21 pump package.
The payload heat exchanger is here, I believe.
Mid body cold plates.
Aft cold plates.
Each radiator side has its own flow control system.
The evaporative heat sinks, one is the flash evaporator that I've shown you a couple times before.
I will describe it a little better in the minute.
It is here.
It uses water.
There is an ammonia boiler here that is also an evaporative heat sink.
And when we're on the ground there is a GSE connector for the GSE heat sink.
[AUDIENCE QUESTION] More than you would ever know.
In fact, this is the most complicated, integrated thermal test ever run at JSC.
And I will show you a picture of it in a minute.
We actually tested all of that together.
And it all had to work together.
And it gets worse as we go along.
I will show you all the integration factors as we go along, but it changes from mission mode to mission mode.
It changes within mission modes as to how the system works.
And I will try to describe that.
OK, so this sort of gives you the feel now.
Keep in mind, geometry is going to play an effect here as to how you plum the system up.
So Freon is running through all of those lines there to the radiators?
It will be a little clearer in a minute.
This is a schematic.
Let's start at the radiators.
On a good day, this is doing the job for you.
All the radiators are getting you nice cool liquid.
The radiator uses a bypass system, so you will see the bypass around the radiators.
It is good to remind yourselves that heat load can be tremendously different.
And the high level mission phases, a lot of electronics, a lot of crew activity, the vehicle is alive and pumping out the power and, therefore, pumping out the heat.
If you ever did one of those 28 day missions we talked about, which was a spec requirement, you can imagine this vehicle is almost quiescent.
It is doing very little.
You're trying to link them the mission.
So you're doing hopefully experiments or something in SpaceLab.
You're doing something else.
The vehicle is not working for you very hard.
Just as an example, we have five computers.
And we use all five during ascent and entry, the active phases for safety, but once you're on orbit, when you have a long mission like that, you actually power down all but two of the computers.
That is just it.
And there are a lot of other systems which you power down.
And that way you're limited in missions by the electrical energy which is limited by the amount of hydrogen and oxygen you can carry for fuel cells.
So, by powering down, you extend your mission.
And, of course, you will also reduce the heat load. And, when you reduce the heat load, you've got to find some way to make that same radiator work for you at a very low heat load.
So it has the capability of getting rid of a lot of heat.
If you don't want it to get rid of that much heat, because you don't have it, then you have to go to bypass system.
Anyway, the next thing in the loop is the GSE heat exchanger.
On the ground you don't have radiators working so you use the GSE heat exchanger.
Then there is the ammonia boiler.
When you reenter the ammonia boiler gives you the ability to operate with an evaporative heat sink prior to the connection of the GSE, and so it is part of the thermal control system.
The next is the flash evaporator.
And you'll notice there are two flash chambers here.
One is called the high load and one is called the topper.
And I will talk about that a little more later.
Then the nice cool liquid, however it was cooled, all these cool it.
However it was cooled is then available for the vehicle.
And there is one more little heat load, and that is the cryogenic oxygen that we're bringing in.
We also warm it up with this coolant loop.
So the flow then goes to the aft cold plates.
And then the flow also goes to the cabin heat exchanger and the payload heat exchanger.
You notice these are either or.
And that's because sometimes you don't even have a payload heat exchanger use so you don't use it.
Sometimes you have a lot of use for it.
And maybe, for example, SpaceLab and Space Station have kind of an arrangement.
You wouldn't be in the cabin as much so you can divert that.
It allows you to use the capacity in either of two places here.
Then you come on down and come through your pumping package, there are redundant pumps.
Then you go the fuel cell heat exchanger because now you've used the nice cold fluid up.
And so the fuel cell can stand the warmer temperatures.
Also some cold plates.
Now, it might have occurred to you, it occurred to me, why did we waste our nice cold fluid on these cold plates?
Why didn't we put those cold plates down here so we could have had more cold fluid for our cabin heat exchangers?
And the answer is geometry.
Those are way in the back.
The cabin is in the front.
After you've come forward from the flash evaporator, which is in the back, to the cabin, you don't want to go back to pick this up.
You wouldn't want to have to go back to the back of the vehicle and then back forward again, so this is a geometry problem.
We have cold plates here.
We also have cold plates here.
They come forward.
The hydraulics heat exchanger is the last thing on the loop and then back to the radiators.
We will talk about that a little more.
The functions of the active thermal control system.
Collect the waste energy from all the systems.
We just talked about that.
And rejected radiatively to space.
That is our first choice.
Our first choice is to use the radiators.
It turns out that the radiators in some heat load environments don't do the job.
You have a high load and a bad environment, you cannot get rid of all the heat.
Also, we are producing a lot of water, so something constructive has to be done with this water.
The fuel cells are pumping it out because every time you make a kilowatt you've got more water to use.
So we decided to augment the space radiators with the evaporative heat sink at these high load cases.
This gives us an ability to accept a radiator system that cannot really do the job by itself but make it completely adequate for some of the low heat load cases, but in the high heat load cases then it is augmented by water.
Now, when you want to get rid of water and you don't have some of this bad environment you can actually throttle the radiators down.
And, if you throttle them down, then you force the evaporative heat sink to come online and do the job for you.
In other words, you change the set point on the radiator.
Normally we use 40 degrees set point.
If you change it to 56 degrees and let the water take you from 56 back down to 40 then you artificially get rid of water.
It is a good water dump system.
During ascent, over 100,000 feet we use the water boiler.
The flash evaporator.
Not a water boiler.
That's a Freudian slip, I guess.
The Apollo had what's called a water boiler.
It was a nightmare.
We said never will we do that again, so we ended up with a different design for the Shuttle.
The reentry system below 100,000 feet is ammonia.
It will carry you all the way to the ground and on the ground until you get the GSE.
Water won't carry you because water won't evaporate less than 100,000 feet, but the ammonia will so that's the reason it is there.
Let's talk about the radiators themselves.
The two payload bay doors are a mirror image of each other.
They are radiators on each side that are exactly mirror image.
I don't know what happened there.
Anyway, this is supposed to say that the radiators on these two sides are mounted and are plumbed in two separate cooling loops.
The reason for that is there is always a redundant loop.
Obviously, you have to be able to take a failure and still have a good system.
If you run both loops through both radiator sides and you took some sort of collision hit then you could wipe out both of your cooling loops in one hit.
And so that is unacceptable.
By plumbing the systems up with mirror image radiator systems on different loops then you can still use the capacity together.
But if you lose one side you still have half your capacity left.
And plus you have your evaporative heat sinks to make up the difference.
So we can survive with a door out.
I mentioned the dual set points before.
I also mentioned the bypass thermal control.
I mentioned the dual set points, 40 and 56.
40 is our normal.
If you're trying to burn up some water you go to 56 and you use the difference in water.
The radiators, we have two different kinds of radiators.
We have the single-sided that are on the back of the vehicle and some two-sided ones on the front of the vehicle.
The reason for that is when you open the door you expose the underside of the door.
If you have radiators on the underside of the door then you've exposed your radiators.
But if you lift the radiator panel off the door, because the doors go way down, then you can see the underside.
It's not as good, you don't get a full view of the heavens, but it does get rid of heat.
And so we needed that extra capacity.
On the front four panels they are the two-sided and on the back four they are single-sided.
The radiators themselves are made out of a honeycomb aluminum structure.
The tubes were imbedded in the structure so that the surface was smooth, and that was because we wanted to use a silver Teflon tape for our thermal control coding.
For previous vehicles we always painted the radiators, painted them white.
If you look at the alpha epsilon white paint, it is pretty good.
I think Apollo was 0.18 alpha and epsilon about 0.92.
That's pretty good.
The market had moved along.
Technology had advanced and there was not the silver Teflon out there.
And what the silver Teflon does is the silver gives you the reflection which is good and the Teflon gives you the radiation which is good, so you get the best of both worlds.
Silver Teflon was about 0.05 epsilon and about 0.88, 0.9, something like that.
You lost a slight amount in epsilon and gained a lot in alpha, so basically you could ignore the sun which is what you'd like to do, is ignore the sun.
It turned out the silver Teflon had some issues.
We tested the radiators.
The radiator was not a slam dunk with eight panels, four of which are two-sided.
Effectively you have 12 panels.
You have fluid going through those in parallel tubes.
You're trying to guaranty that the fluid all goes where it's supposed to go.
And, therefore, the viscosity of Freon, which is very low, analytically said it was all right.
But we wanted some testing to prove it so we did test the entire radiator system.
And, in that test, all the silver Teflon fell off.
It is a really good thermal coating but it doesn't seem to be very practical.
It turned out that the problem was adhesive.
We did a little adhesive work and the materials people came up with a Permacel that worked perfectly.
They have never come off again.
In fact, we get 10, 12 years out of the radiators, lots of time.
The problem that the Permacel couldn't solve was beneath the tape you could get very small little out-gassing of the materials.
Almost all materials outgas some.
Basically, we would put bubbles underneath the tape.
We went to a perforated.
There are millions of little holes in this tape.
And it is placed on the vehicle and it stayed on just fine.
It wasn't very long, though, that people began to worry about the focusing of this silver, that the doors are curved upward.
And so there is a focal point there that is a very, very hot point.
And there was concern that that's really not a good thing to have.
If we could make the surface less specular and more diffuse without losing the alpha and epsilon we would have a better design.
And so they dimpled it.
Now we have Permacel dimpled, perforated silver Teflon tape.
It works great.
The flash evaporator.
Water is the flash evaporator's evaporant.
This was a brand-new design.
The water boiler on Apollo was no good.
It was a problem.
The flash evaporator is a very simple concept.
You have a chamber.
You run your coolant around the chamber that you want to be cooled.
And you spray water on the walls of the chamber.
And, if the pressures are all right, the water will flash.
You won't get ice.
It will go straight to steam.
Steam will go overboard and it will all be wonderful.
And if you can convince a program manager of that good luck.
Anyway, the way to convince a program manager is to show him good test data.
During the development, we developed this process of spraying the walls and not getting water carryover which will obviously freeze up your steam duct.
Which you don't want.
But, as the design evolved, we had to get more sophisticated because we needed this flash evaporator to burn up the extra water when we didn't need the fuel cell water.
And we did that with that 40 to 56 set point.
And so we needed a small evaporator that could handle that.
It was called the "topper." It tops off the radiator.
But when the doors are closed there is nothing else to get rid of your heat, now you need a loss of capacity.
And so the second chamber was the hallowed chamber.
Together they do the whole job.
When the radiators are working only topper, you can come home with this system functional and make it home.
It turns out we never did freeze up the steam duct so that part was good.
It turns out that we had some freezing in the chamber.
And, in fact, we had to develop a process that acknowledged that that could occur.
And how would we flush it?
It is called "flushing." And so we did develop a process.
And it has never failed.
The flushing process always works.
And we have had on several occasions, I probably cannot count them for you, but four of five times maybe over the Shuttle flights we've had some freezing inside the cavities.
There is a non-propulsive overboard vent.
That was the one going out both sides for the topper because it could be used for very long times during the mission and you might be pointing or whatever you might be doing.
But when you're coming home the high load is just a big out the side of the vehicle type duct.
The ammonia boiler, its heat sink is ammonia.
As I mentioned before, it evaporates all the way to the ground where the water won't.
The redundant boiler is here.
Up here there are no redundant boilers.
These two chambers have redundant everything.
They have redundant feed water systems and redundant control systems, redundant spray nozzles, redundant everything, but the chamber is a chamber.
You get what you get.
They are redundant boilers is the way the ammonia boiler gets its redundancy.
It was designed to be utilized during entry and on the runway until the cooling is available.
It turns out that we don't do that anymore.
It turns out operationally we learned that if we cold soak the radiators before we shut the doors and then bypass them and come home on the flash evaporator that there is a lot of heat capacity still left in those radiators.
There is a lot of fluid out there and it is cold.
And so we can actually go all the way to wheel stop on the radiator.
And so at 175,000 feet we start flowing the radiator.
And at 100,000 feet the flash evaporator is off and we cold soak all the way home.
And after wheel stop the ammonia boiler cranks up.
I mentioned the Freon 21, the low viscosity.
We got an excellent performance out of that.
The low viscosity is really, really important, as much parallelism as we have.
I didn't really give you the numbers.
In the single panels we have 26 tubes.
In the two-sided panels, I believe, there are 66 tubes.
Lots of tubes.
Lots of parallelism.
It is awfully easy to get flow instability in a thermal system like that but the Freon loop does great.
We have redundant pumps, all liquid heat exchangers and cold plates.
This is that chamber that I told you.
If you can look right down here, this is one person.
In fact, there is a radiator panel.
This was during buildup.
Twice we tested the flash evaporator in this environment once as part of the integrated system with all the radiators and once by itself.
And twice we tested the radiators, one as part of the integrated system and once by themselves.
So we did essentially three large tests.
And the one that had the entire system in it, had all the cold plates, had everything in it, as I said, is the most sophisticated thermal test we've ever done at JSC.
And it proved that the system could work.
It also proved that we had to tune the flash evaporator nozzle because it was wrong.
But we did get the data, we did prove it was wrong and we got it re-machined.
A machine out of columbium, somebody else will have to answer why.
And so it was a problem for Aaron to go get us another one, but it fixed it and it was successful.
[AUDIENCE QUESTION] You can have a command module and a service module and a stack configuration in side that chamber.
And it had a solar and full vacuum.
[AUDIENCE QUESTION] It was a manned chamber then.
It is not now.
After Apollo-Soyuz, it hasn't been manned since then.
It's still available for testing but is not manned.
It has a sister chamber 100 feet that way where all of the EVA testing is done.
A good example, I think, of how the Shuttle Program built on a lot of Apollo-- The same thing at the Cape where a lot of the launch hardware was adapted is the Shuttle Program, you know, you heard about the budgetary constraints.
The Shuttle Program never could have afforded to build something like that. But luckily a lot of this equipment was built during Apollo when the money was much more available. If you have been keeping count, we're getting toward the end here.
I did want to mention, before we left the main orbiter systems, that there was some rotating equipment life testing that we did for the program.
The equipment we're talking about here is the cabin fan, the water gas separators, the avionics bay fans, cabin coolant and the vehicle coolant pumps.
The life requirement was 100 missions, and that was calculated by somebody to be 20,000 hours.
If you could show 20,000 hours of successful operation, you had the design life of the hardware.
We set up a laboratory to run the equipment.
It was obviously a low-maintenance lab.
We collected data and checked in the room occasionally to see if everything was all right.
It turned out that all the equipment passed the 20,000 hour requirement.
We did learn something, though, that some of that stuff was so noisy.
Oh, it was noisy.
There was some work done on some muffling, but the hardware itself was all good.
We are at the end.
Yes.
Were there noise requirements [AUDIENCE QUESTION]?
Yes, there was a cabin noise spec.
And, yes, it had to be met.
And I don't remember how it was met.
We put mufflers on some of the equipment.
Maybe some insulation inside of the ducting, I'm not sure.
If we have time, COHEN: I will tell a little anecdote here.
I chaired the Change Control Board.
[NOISE OBSCURES] The medical organization came to see me and said that the cabin was very, very noisy and it was going to really do a detriment to the astronauts.
And it was very expensive in terms of dollars and weight to really get all the cabin fans and cabin environment down to the level they wanted.
I thought for a moment.
I go out to California a lot.
I go out to Rockwell and stay in motels that are on the Los Angeles freeway.
And I said you don't see me worried about my ears in staying in those freeways and not being able to sleep and having all that noise.
And the doctor looked at me for a moment and said, well, you're already deaf.
And I said what did you say?
But we did have to make some modifications of it to actually reduce noise.
Let me interject one item.
I think somebody asked the issue about the IMU.
We decided to pick the IMU off the shelves, and it was air-cooled, to really do it right.
If we would have done it right, we would have picked an IMU which was compatible with liquid cooling rather than redesign it.
But we decided to stick with the IMU that was air-cooled.
Whether that was the right decision to make, I don't know.
It seemed like it was because I think we've only had one problem with an IMU.
I don't think we've had many problems with the IMU.
But that is a little anecdote that I thought of.
That's a program manager's trait.
Probably the air-cooled IMU was a less expensive option.
It might have cost a little in terms of thermal efficiency, but that is only energy power.
And power was available so it was a good tradeoff.
This is the last of the subsystem areas.
This is the EVA airlock support.
The Environmental Thermal Control and Life Support System does support the airlock.
It maintains the cabin pressure and composition during the airlock repress and depress.
This is really a requirement back on the ARS and the pressure and composition control system because airlock use basically dumps cabin gas and then you repress the airlock.
It is a requirement that you cannot upset the cabin while you're doing the EVA function.
We also provide the service and cooling umbilical.
If you have any kind of mental picture of being in the airlock, the crew is connected with cooling and oxygen through an umbilical so they don't use the consumables in their spacesuit.
And so that is provided by the ETCLSS.
Heat rejection, the crew wears a liquid cooling garment.
And the cooling for that is done by the vehicle until you go EVA, of course.
Then the recharge.
We supply the backpack O2 recharge from the 900 PSI cryo.
We supply the water to recharge for the sublimator.
NASA is overrun with acronyms.
EMU is extra vehicular mobility unit, which means nothing but spacesuit and backpack.
So the spacesuit and backpack uses an evaporative heat sink and it uses a sublimator.
And the idea there is it's a device that exposes a layer of ice to space and then it sublimates and you get your cooling from that as opposed to putting a liquid water on a surface and flashing it.
Anyway, that has to be serviced.
That water is provided from the system.
And then the condensate that the crew brings back, EVA, that's a sweaty job.
That condensate goes back into the system.
So the airlock support is another part of this system.
In fact, that is the sixth part.
So we are through.
This is a picture of the airlock.
Not really the airlock.
This is the test chamber that we built to put the equipment in.
You'll notice it has the right hatch in it.
And, if you can recall back an hour and a half ago, I showed you a picture where there was this hatch basically on the backside of the test chamber.
We hook those together.
Because, as I said, as you depress and repress the airlock it affects the cabin.
So we needed a test environment that had both those simultaneously.
But the difference is this has to go all the way to vacuum.
So we connected this to our vacuum system.
And, when the crew is inside this, it is a manned vacuum test.
And when the crew is inside the other test chamber it is basically sea level or, at most, 8 PSI would be the lowest it would ever be.
And I think the last picture is a spacesuit inside the airlock.
And this is just a little cutaway showing the airlock.
This was mounted on the back of that chamber you saw earlier.
If you bring that picture back up, I will talk to that for just a minute and then we will have some questions.
Before every flight, one of the things that we do is that every EVA crewmember takes his or her spacesuit into that EVA test chamber, into the airlock test chamber so you actually get a chance to take your own suit down to vacuum.
And particularly, before you do your very first EVA, it is really a confidence builder because the physical sensations that you get in a suit -- And remember you're at about a 4 PSI pure oxygen environment.
Your body, the inside of your mouth feels a little different.
There are funny sounds with the fans inside the suit.
The sorts of things that you don't want to experience the first time when you actually go outside into space.
It is much better to do it here in a controlled environment where if there is a problem -- Actually, you are supported because of the weight of the suit.
It doesn't look like there is anybody in this suit.
But, when you're in this suit, you're actually supported by a cable to the ceiling of the chamber which is on quick release bolts.
You're suspended up here.
And, if you ever have a problem, they can release this, repressurize the chamber within about 30 seconds.
I think that's the limit for a manned rated chamber.
You're going to be able to repress in about 30 seconds and then just lift you out, suit and all from the top, and get you out of your suit and get you to the medics.
This is just an inverted bell jar.
The hatch is not sealed.
When you depress it just like pulls it out and seals due to the pressure difference.
You just lift the top off.
Yet another example of the extensive testing that we go through before every mission.
I might add one more thing, which I forgot actually to add earlier.
The manned rating is a term used in manned spacecraft which means that you've specifically tested it and said that its design is worthy of supporting a human.
The airlock had to provide that function.
So Aaron was faced with man rating the airlock.
And manned test capability is very scarce.
In fact, JSC may be the only place anymore that can do it.
Anyway, we volunteered to do that as part of our test program as a programmatic requirement.
Not as testing or watching or confirming anything, but as a prime part of the test program was the manned rating of the airlock.
And that was done in Houston.
That is an interesting thought.
If my memory serves me correctly, one of the problems we had in the early part of the Manned Space Program was doing some chamber runs McDonnell-Douglas with a two-gas system where we actually lost the heat [NOISE OBSCURES] in the chamber and almost lost a test subject.
And that was when Apollo decided to go to 100% oxygen.
And that is what led up to the fire on the pad.
reason. And that is really if you can realize that 100% oxygen at 16 PSI everything burns.
It went to 100% oxygen at 16 pounds per square inch with an invert over the hatch for another
Stainless steel is not self-extinguishing at 16 PSI.
And, of course, that's what we were up when we had the Apollo I fire on the pad.
It was a function of doing some test work with the combined atmosphere.
So you have got to be careful that sometimes you don't over-react the thing.
Then we went back to a different type of atmosphere.
Did you have a question?
The systems you've been describing today seem to take up a lot of space in the Orbiter like other systems.
How did you negotiate and manage other subsystems for the space and the volume in the way that you wanted?
That is a good question but you're asking the wrong person.
We are the government.
We hired a contractor to design the vehicle.
Now, the design aspects of it, the philosophy of it, the functionality of it we were very, very integral with.
We watched, we audited, we said that looks smart, but we didn't go and say put that cold plate here and put that heat exchanger there.
The vehicle integrated design was Rockwell's.
Let me tell you how it was done.
Actually, in those years we didn't have CAD/CAM systems.
What we did is used everything on mockups.
We did mockups.
The system was laid out of what to do and the functions of what they had to do and the plumbing.
And this was all laid out on a mockup.
And basically that was how it was negotiated in terms of what you need.
In today's environment you wouldn't do that.
You would use electronic capability.
You would use your CAD/CAM systems.
You would actually draw it up on your computer.
In fact, the 777 was built that way.
The 777 at Boeing, they didn't use hard mockups.
They used electronic mockups.
And that is what we would do today.
And it would be much easier.
You would probably call all the engineers together.
You would sit down and show how the equipment was laid out, get a satisfactory buyoff on it and do it much more rapidly and much more quickly.
In the future, what you will be doing is actually use a CAD/CAM system or CAD system to actually negotiate the space you need for it.
That is a good question.
And it was based on purely that you would get drawings, mockups, go out and lay it out in a soft mockup or hard mockup.
You would get the engineers to come out, the manufacturing people to come out and say this is how it is going to look and then get a buyoff on it.
Today you wouldn't do it that way.
You would do it all electronically so it would change.
Well, let me ask you a question.
Of all the work you've done in the Shuttle what was your biggest concern in getting ready to fly or during the flight?
What did you have the biggest concern about?
I was young and confident.
I don't know that I had a specific area that I was really concerned about.
Like these people right here, right?
Young and confident.
I did recognize, though, but it did result in the integrated tests that I showed.
I did recognize that we had the most highly integrated thermal control system ever conceived.
And it had, as I said, modes within modes.
It changed modes with respect to the vehicle phases.
It changed modes with respect to operation.
We had 9 PSI that went to 10.2 PSI.
We had 8 PSI.
We had thermal control loops, ammonia boilers, flash evaporators, so I did recognize the sophistication.
In fact, we proposed early on to use that large chamber in an integrated vehicle test.
Not we, me, but the big we looked at an integrated test like the Command Service Module was tested.
And that was viewed to be impractical.
It was cut it off at the bulkhead.
You could do the forward cabin part and then do the aft section, but that was viewed to be impractical.
But it still was a very integrated design.
All the systems engineering that was there created that inner-dependency.
And I think I was smart enough to know that there was certainly some potential risks there.
Remember in the very beginning of this I talked about ownership of all the technical aspects?
We had ownership.
We believed that what we had done was prudent, appropriate, and we believed it would work.
It wasn't like you bought something and you hope it works.
Can you say a word of what you think, this is looking in your crystal ball, about the CEV?
Do you think it's going to have a very similar type system or have you thought about that?
Well, we hope so.
Our new administrator has sort of back to the grassroots philosophy, or at least right now.
And so we hope that the CEV will be developed with a lot of government involvement, that the government will have ownership again of the technical aspects of the design.
If that happens then I think a lot of the experience that is around and a lot of the free-thinking and the independence that the government can bring to that, there is another school of thought that says keep the government out of the way and let the contractor go do their thing.
And, obviously, you wouldn't get that position from me but there are people that believe that's the right way to do it.
Do you think the system will look pretty much like this or similar to it?
Well, similar, yes, I do think so.
I believe that the very tight schedule for the CEV is going to put a lot of pressure on the program manager to go with the tried and true.
So I don't think there is going to be innovation that has risk with it.
Maybe there will be innovation that is good engineering, but I think the innovation with risk will be very much minimized.
Because taking Shuttle offline in 2010 there is going be a lot of pressure to get this vehicle finished.
I think that is going to breed some conservatism on the design side.
We talk about considering operation while you're doing the design, but there were always things once the design was made.
Well, like you mention, once we came up with a requirement for a 10.2 cabin we had to develop workarounds.
And one of the other things that after the system is designed, we then start to figure out how can it fail and work on malfunction procedures.
And I know that the malfunction procedure for loss of two coolant loops that was probably the worst simulation case that we ever had to deal with.
I am curious to get your opinion.
Walt described how we have two independent cooling loops so that in principle one failure won't take them both out.
Now, I don't remember what it was, but they did discover a single point failure somewhere in there which I think was corrected but where you could have lost both of the cooling loops with a single failure.
The only failure I remember is one that John Young used to worry about.
It had to do with if you did lose a radiator that that could bleed your accumulator down.
And so they did add an isolation valve so they can isolate the radiator side.
But the idea was to obviously not lose your fluid with a single hit.
That even though you have a nice completely functional thermal control system it is useless because it has no fluid.
We did have an emergency de-orbit procedure for loss of two Freon cooling loops.
And, of all the things that we practices, that was the hairiest because it was absolutely time critical.
At this point, you have no way of getting heat out of the cabin so you cannot ultimately cool your electronics.
The cabin gets hot, your electronics get hot so you have to do an immediate de-orbit.
Hopefully you're within range of a landing site.
And then you turn off all the equipment that you can possibly turn off, including most of your computers.
And, when you're riding down through entry, you basically watch the temperature of the
computer. And, as each computer would get up to its failure temperature, you would then switch on the next computer, transfer the data, you know, turn off the computer which has just about failed, and hopefully you could make it to the ground.
And I know the instructors that would lead us through this exercise said that the information they had gotten from the thermal analyst was that nobody was really sure whether we could make it.
I don't know what your opinion was or not.
We practiced it.
But obviously, I think I've told you, nobody has ever died in a simulator.
But you never know whether the thermal model that they use in the simulator is really going to duplicate the way the Shuttle is going to behave in an absolute emergency situation like that.
We survived in the simulator but I don't know what your feeling is.
Well, we do address the thermal model accuracy.
We do specific testing to validate the thermal model so we do have pretty good confidence in those.
But the real issue in overheat is that when does a piece of equipment stop working in an overheat situation?
I mean there is no magic number.
It is not like at 143 degrees it is OK and 144 it is not OK.
And it varies with age, it varies with equipment and it varies with a lot of things.
So there wasn't really a precise answer as to how long you could keep everything functional and come home.
Yes.
You talked earlier a little about oxygen and nitrogen mixing in the cabin.
I was wondering if there is a way to really study that on the ground since you're not in a DoD environment.
How do you know there are not pockets of nitrogen sitting around that an astronaut can stick his heat into?
Very good.
I just forgot to mention that earlier.
The testing that we ran, we had to set it up very carefully.
If you notice, there is a flight deck and a mid-deck, so obviously buoyancy is going to mix that stuff for you.
What we did is set up a thermal condition where less than one degree thermal gradient everywhere in there had to exist before we ran the test.
And that was to guaranty that we had no help from the naturally induced circulation.
But you are absolutely right.
That test would have been a useless test without neutralizing the gravity effects.
And actually the first time that I did the spacesuit test in there we actually used the 10.2 protocol.
So I actually had to go in the day before, take the cabin to 10.2, spend the night and then we could do just a 40 minute prebreathe.
For most of the other tests, when you go in, in order to avoid the inconvenience and expense of staying through the night, you go in early in the morning.
And then you're coming right from a sea level environment.
So, before you go down to 4 PSI in the suit, you actually have to prebreathe pure oxygen for four hours.
As Walt said, that was the alternative.
You basically just get in your suit.
You can either bring a book and read your book or they will pipe music into you if you want to give them a CD, or I guess there was an audiocassette in those days, or they have a little black and white TV monitor.
And you just sort of sit there for four hours.
And the problem is you're not allowed to go to sleep.
That would be the easiest thing, but they want you actually to move around because when you're moving your muscles you're actually then doing a better job of de-nitrogenating.
So you have to stay awake.
And if you don't say anything for more than about 10 minutes they will pipe something in and say, Jeff, are you awake?
But this was a very, very useful facility.
I mean they used it for all different sorts of things.
And I guess that was the one new facility which was built for the Shuttle specifically.
Jeff mentioned several times the 10.2 and I talked about the 9.
Yes.
The 9 was the original design, but the issues with the 9 PSI had to do with the oxygen percentage got too high.
And so there was much concern about flammability.
The idea was if you could raise the pressure up some to 10.2 then that would be less of a flammability issue.
But then the problem became at 10.2 that the suit at 4 PSI was too much of a drop.
If you're in the bends while diving, somebody mentioned diving earlier, scuba, you could usually use a two to one.
If you don't reduce pressure more than half you're probably OK.
You're probably not going to get the bends, but half of 10.2 is not 4.
And the suit is at 4, so there was that issue again.
They raised and bumped the suit up a little bit and got it up to 4.3 and went to 10.2 and developed the prebreathe protocols that made that acceptable.
That is sort of how it balanced out.
That was another operational move later.
And, as Walt said, you're spending enough time, once you get in the suit and you purge all the nitrogen out, and so you're sitting in a pure oxygen environment, you've got to do a bunch of tests and check out communication tests and cooling tests.
You basically are not losing much time by having to spend 40 minutes prebreathing.
The whole thing worked out pretty well.
And it will be an interesting question on how they designed the CEV because the CEV has to be compatible with docking to the Space Station which works at a sea level environment.
But, on the other hand, if you're going to take the CEV into a situation where you want to do a lot of space walks, you probably want to be able to operate it at more like 8.5 or 9 PSI so that you can go right into a spacesuit without an extensive prebreathe.
And, of course, people are always trying to figure out could we design the spacesuit to work at a higher pressure?
But then you give up maneuverability.
We will talk about that in another lecture that I will be giving on EVA systems.
Walt, thank you very much.
I enjoyed it.
[APPLAUSE]
Free Downloads
Video
- iTunes U (MP4 - 256MB)
- Internet Archive (MP4 - 410MB)
Subtitle
- English - US (SRT)