Flash and JavaScript are required for this feature.
Download the video from iTunes U or the Internet Archive.
Topics covered: Molecular Description of Acids and Bases
Instructors/speakers: Prof. Christopher Cummins
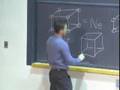
Lecture 19: Molecular Descr...
Related Resources
The following content is provided by MIT OpenCourseWare under a Creative Commons license.
Additional information about our license and MIT OpenCourseWare in general is available at ocw.mit.edu.
Today is a very big day for all of you.
Your first semester at MIT is half over.
And, in addition to that, you have a lot of challenges remaining ahead of you for the rest of this semester.
I am going to help make the chemical challenges fun for you.
That is what I am here to do. I love chemistry.
I love teaching 5.112. This is the best part of my week. So, here we are.
Do you guys know that chemistry can be incredibly fun?
Let me just show you how fun chemistry can be.
And that is just the gravy. The fun part is actually making the molecules. Any of you see this picture in U.S.A. Today recently?
Not reading that important journal, I see.
Well, this picture did appear very recently in U.S.A.
Today in a story that talked about the recent Nobel Prize in chemistry that was awarded to Professor Richard R.
Schrock, of our department. And, in fact, I don't know if you realize this, but Professor Schrock was the first person ever awarded the Nobel Prize in Chemistry at MIT for work conducted while at MIT.
That is a pretty amazing accomplishment because we have so many tremendous chemists on the faculty here, and he is the first one to break through in that manner.
And maybe you are here at a very special time, and we will see a number more of these in the next few years.
May with you contributing, so much the better because you are here. And let me just explain something, too. Chemistry is fun.
And only on very rare occasions like this would Professor Schrock be drinking champagne at 8:30 in the morning, when this picture was taken. The Reuters reporter, Brian, who took this photo, is actually a pretty good friend of Professor Nocera here. And so he rode over from the Schrock household. And Dick and Dan came in together in the same car, as they often do, from Winchester on that morning, which was a very exciting day. If you come to see me for office hours maybe I will tell you more about it in detail, but it was an exciting day. And I am in here, too. My name is Kit Cummins.
Kit is my nickname. My name is Christopher.
You can call me Christopher or Kit, or you can call me Professor Cummins, or you can just say hey, chemistry guy. That will be fine.
I am in this picture primarily because it is part of being in the right place at the right time, just like I am here now in front of you. But also I am in it because we three are three in one of the areas in our department that really focus our research on inorganic chemistry.
And what is inorganic chemistry?
I will say more about this during the semester.
But inorganic chemistry is really the chemistry of the elements of the Periodic Table inclusively.
So, a pretty broad topic. And I like doing chemistry with lots of elements. And so that is why these guys and I share an association with each other.
And, in fact, I was a graduate student with Professor Schrock. And I am very, very proud to be able to say that.
Hopefully, my computer will be staying on, here.
And I want to point out, too, that people who love science want to know something about its history.
And part of all of that is knowing the academic family from which you derive, depending on the person you've studied with in learning about chemistry.
And I studied with Schrock. And Schrock studied with Osborn, a fantastic chemist in his own right, no longer alive, sadly, to see his progeny, Professor Schrock, win the Nobel Prize.
And Osborn studied with Wilkinson, and Wilkinson was also a Nobel Prize winner. So, you do not have to go very far back in that lineage to encounter that recognition, the Nobel Prize, Schrock, and then it skipped Osborne, and if you go back to Wilkinson, there is another one.
And you can keep going back. And I am not going to go through that whole history today because I have another history that I am going to need to go through with you today.
And I am going to bring this back over so it does not distract you. And this other history that I need to impart to you today has to do with some of the contributions of Gilbert Newton Lewis.
This is one of the most famous of all chemists ever.
And if you like chemistry, I encourage you to think of the word Newton, as it is found engraved in one of the pillars in Killian Court at MIT as the middle name of Gilbert Newton Lewis. It is conceivable that having that middle name weighed heavily upon Gilbert during his lifetime. But it remains a good moniker.
And in the future, when you have kids, I encourage you to consider using it as a middle name.
It worked well in this particular case.
When you get the notes for today, which incidentally will be available on the website after class today, so you don't need to write down everything I am writing down.
You are actually going to get a little bit more in the online version than what I am writing down here.
And if you find that you forgot something that I said during class, just email me. I will tell you what I said.
It is not a problem. But if you want to write down notes while I am speaking just to make notes that you can refer to later to refresh your memory, that is fine.
I think your taking in information in lots of different ways. We are going to use the computer and we are going to use the blackboard today.
And you are going to have available stuff from the Internet a little bit later, too.
And all of that is good. I think you should try to reinforce and not worry if some of the information is a little redundant. And never be afraid to ask questions, especially, I like to take questions by email or if you come knock on my office door.
In 1916, Gilbert Lewis published a landmark paper in chemistry in the journal of the American Chemical Society which remains the flagship journal of the American Chemical Society.
And this paper was entitled "The Atom and the Molecule." Besides being an incredibly important and influential paper in the history of chemistry, "The Atom and the Molecule" was also the basis for -- Like I said, besides being a hugely influential manuscript, "The Atom and the Molecule" also laid the foundations for Lewis's thinking about electronic structure theory that shaped the way we still today talk about valence and chemical bonding.
So, an incredibly important paper from 1916.
In the lecture notes today, you will find the specific volume and page number for that reference in the journal of the American Chemical Society. And I encourage you in your copious free time to download that paper.
It is really great. All the American Chemical Society journal articles are available, from the very beginning. And you can download it and read it. And, having had this introduction today, I think it will probably prove to be a very fascinating exercise for you, if you choose to do so. It also was the basis of a book that Gilbert Newton Lewis later wrote on valance and on atoms and molecules. That is also a classic in chemistry. And, should you be shopping for chemistry books on e-bay or something, you might find a rare copy of it. And if you do and choose not to buy it, please alert me and I will buy it.
But Gilbert Newton Lewis, "The Atom and the Molecule." In this paper you are going to see, if you do choose to download it, that he was thinking about polarity.
And he was also thinking about the relationship between the polarity of a molecule relates to the polarity of a substance.
And I mean, when I say a polarity of a substance, that that would be a sample, you could say a water molecule down here and liquid water up here.
And you will see this kind of sigmoidal graph that Lewis made in thinking about how polar condensed phase substances were as compared with the structure of the molecules that comprise the substance. Down here, in this region, you will find that there are substances that are not terribly polar. And what I have written here is the organic shorthand for the hexane molecule.
Liquid hexane is an alkane, akin to methane, which is natural gas. And so, if you are not familiar with this way of writing organic molecules, I will just tell you briefly that this terminus is CH three or a methyl group, and then it is CH two, CH two, CH two, CH two, and then CH three, another methyl group.
So, that is some organic shorthand.
And I will go through that kind of shorthand occasionally so that you become familiar with it.
And that is the hexane molecule, a very non-polar molecule composed of elements, carbon and hydrogen, that have very similar electronegativity.
And then he goes on up the curve here, and you find this familiar molecule, benzene, considered next, as being somehow more polar than hexane, and liquid benzene accordingly in its solvating properties in the condensed phase, being a more polar medium, as it were.
And then, as we continue progress up this scale, he writes next molecules like the diethyl ether molecule.
No doubt you are familiar with ether and its applications as an anesthetic. The first use of ether as an anesthesia in an operation in medicine was carried out just across the river in Boston. There is this historical Ether Dome there that you can go and look at.
But, nonetheless, we are just getting up a little bit more polar than benzene. And then next you find molecules like this one. That contains an organic functional group called an ester.
That is ethyl acetate, which is a substance that you might have smelled if you ever built models, because some of the glues that you may have used would contain it, or the paints. And so, it is a system that, in terms of its molecular structure, is more polar.
And, when you use it in the condensed phase, it behaves in a manner that you would describe as more polar.
And then, finally, you get up to some very polar systems, like the water molecule.
Like the ammonia molecule. And, finally, Lewis talks about a species like this, which is sodium twice sulfur. That is actually called sodium sulfide. And it is a salt analogous to table salt, sodium chloride.
And being a salt, it is obviously very polar because there is quite a large degree of charge separation between the atoms in the molecule.
And, accordingly, if you take that salt and heat it up until you have got it as hot as its melting point and make it liquid, that molten salt has individually charged ions moving around in solution.
And that provides a very polar medium, which would be excellent at dissolving polar things. You will notice here that on the non-polar side we have elements, carbon and hydrogen, that are very close to each other in electronegativity.
And then, as we examine this relationship, we start putting in elements like oxygen or nitrogen, which are very electronegative close to the upper right-hand side of the Periodic Table. That introduces polarity both in the molecule and in substances comprised of the molecule. And then all the way over to the involved atoms, sodium and sulfur are very different in electronegativity and, therefore, have very strongly separated charges.
And, in thinking about this, Lewis proposed the notion of a continuum. And his musing on this are really quite lucid in that paper, but the idea was that a molecule, or a part of a molecule even, you can analyze molecules in terms of smaller pieces of them that can be identified within the larger molecule, can lie on either side of a continuum in reference to a number of different properties. And the section of class that we are launching into today deals with acid-base properties.
Let's mention that first, acid and base.
These are two ends of a continuum.
But he also recognized that you could have a molecule that would be an oxidant. And the counterpart to that is a reductant. And then, here is a term that maybe those of you who have studied organic chemistry in more detail than most would recognize.
This is electrophile.
Electrophile has a counterpart called a nucleophile.
And then, the fourth one that I would like to mention is acceptor.
And, of course, the counterpart to that is donor.
Please remember those four pairs because when you can understand and make predictions about those four properties as a function of molecular structure, then you will be a chemist.
I mean that is a large part of what it is all about, understanding all the different chemical properties that will make it interested in terms of acid-base, oxidant-reductant, electrophile-nucleophile, and acceptor and donor.
And the currency of chemistry can be seen, with reference to this, to be the electron. That is what chemistry is all about. And so, we will be talking a lot about atoms and molecules with reference to their properties and how the properties stem from knowing something about the electrons in the molecule.
And I think you are going to find this to be quite fun.
With respect to the properties of the medium, let's talk about a substance like hydrogen chloride.
This is just to illustrate what I was saying over there.
If you take a molecule like hydrogen chloride, and it is a gas, of course.
And if you make a solution of it in hexane, hexane is going to be our solvent, and then what you find is that in this low polarity medium hexane solution, liquid hexane, the HCl molecule remains intact and floats around in solution, interacting weakly with hexane molecules that surround it. But if you take this HCl molecule and put it into a solvent like water, then something on the other end of the continuum transpires because we get ionization to produce hydrogen ions.
And they go off and are separated in solution from chloride ions. And we will talk more about just what is going on when you separate H plus from Cl minus and let them diffuse apart in aqueous solution. But you can see, I think, the dichotomy. Either the proton shares in the electrons of the chloride, or it just pops off and ionizes and goes out into solution. And Lewis wanted to understand this. And, to understand it, he developed a theory. And his theory was the "cube theory." In this part we get to draw some cubes.
And he decided that if you thought of the nucleus, which he calls the kernel, of an atom as being located at the center of the system, then if you had an atom like neon, which is a noble gas with eight valance electrons, that these valance electrons would want to get away from each other as far as possible because they repel each other.
They are all negatively charged.
This could be, here, the neon atom.
Here, on that cube, is how he would represent the electronic structure of the neon atom, with the eight electrons here represented by peach-colored circles, each the vertex of a cube. So, they got far away from each other. And you can see that in the historical archives, some of his original sketch books containing these ideas are there, and now you will know what they mean. You will see that he progressed beyond understanding the atom to understanding the molecule.
And here is one of the molecules that he talks about.
Here we have a molecule containing 14 valance electrons, all arranged in that manner. And this could be, for example, the I two molecule.
And let me draw it in terms of a dot structure, -- -- like that. And there was known at the time, partly because of the contributions of Lewis himself, something called the Rule of Eight.
And that was a reference to this notion that most atoms want to have eight electrons in their valance shell, and it is also something that we call today the Octet Rule.
So, when you see the Rule of Eight mentioned in his paper, it is the familiar Octet Rule. And you can see here that one of the key features is two electrons shared equally.
And when electrons are shared equally like that, you have a molecule with symmetrical charge distribution, charge referring to the electrons where they are in space. And then, he showed that you could think about this another way.
You could consider some kind of a geometric distortion of the I two molecule, like this.
Distorted, not only because of my drawing, but because of the way the atoms themselves are thought to be rearranging in this process.
Notice that what has happened here is that the I two molecule is doing something electronically such that one iodine is starting to pull away from the other.
This one over here is carrying with it the electron that came from over here. And, in that case, what we have is a molecule in which we are developing partial negative charge on the right-hand side and partial positive charge on the left-hand side.
As I was talking up here, I was thinking about what I was going to say here, because the ideal is that molecules, even if they are inherently non-polar like the I two molecule, in their equilibrium structure they can have electron fluctuations that lead to partial charges in the manner shown here.
And Lewis was working beautifully toward the development of a theory like this that would allow the description of electronic structure to be made graphically apparent. And this is something that chemists really like to do, is we like to be able to see stuff. I hope I am going to be able to show you some really cool stuff this semester.
And, in fact, even some really cool stuff before we finish here today. So, that is the development of charge as represented using the Lewis cube theory in an I two molecule. Next, one thing you could do is say, let's just continue this process, and the right-hand iodine with its eight electrons pops off.
That would give you an ionized situation in which you had an I minus floating away from an I plus molecule.
And that situation is drawn in the notes, and because I am running out of space on this board I am not going to draw that one up. Instead I am going to go onto another triumph of the Lewis cube theory, which was its ability to explain different chemical bond orders.
By the way, Lewis never got the Nobel Prize.
He certainly should have. A dramatic oversight.
He was born in Massachusetts. He was a Harvard professor for a while and did not like it, and came to MIT to be a professor for a while. And, although he liked that, that was not far enough away from Harvard, so he went to Berkeley, where he became the department head and was very successful over a long period of time in learning about chemistry and in teaching chemistry.
I will invite you, and you will see this in the notes, too, to go ahead and see if you can find a good biography of Lewis because it is very instructive.
Anyway, here is another molecule considered by Lewis's cube theory.
How many electrons are being shared by the two nuclei or atoms involved? Four electrons shared.
We can circle them here. That is four electrons shared.
Therefore, that is not a single bond.
We call that a double bond. And so, that might be a representation of, for example, the O two molecule. Here we have I two, here we have distorted I two with charges developing, and here we have O two. O two is a very interesting diatomic molecule. We are going to talk about that later in the semester, but for now let's just leave it at that. But then, even though this was quite a successful theory, the cube theory, it has certain problems, certain shortcomings, such as, we can do single bonds with it quite well, and now we can do double bonds with the cube theory, but what do you do if you have to make a triple bond?
That was a problem for Lewis. That should be a problem for you, too. Cubes just don't fit together that way. They have edges and faces, and that is it. And they have vertices.
You can do one vertex. So, triple bond is a problem.
And we know that there are molecules that have triple bonds. And we cannot handle them with the cube theory, so we are going to have to something a little different, for that reason.
But there is also another reason that had to do with just considering the chemistry of light elements like hydrogen and helium. Those elements tend to associate themselves with only two electrons.
And so, Lewis decided, well, maybe the rule of two is more fundamental than the rule of eight, and that we should be thinking about pairs of electrons somehow.
Because he looked at what was known about chemistry and realized that in most molecules, the number of electrons is always even, is always divisible by two.
That is something interesting about two electrons at a time being important. You take that together with the triple bond problem, and Lewis started thinking in terms of electron pairs, and this is where this "electron pair theory" comes from.
Although he spent a lot of time on the cube theory, he was perfectly willing to cast that theory aside in favor of something that maybe was going to work a little better, the electron pair theory. And how does that look?
Well, of course, his initial pictures still had the cube, which just shows that once the human mind fixes on something, it fixes on it with great tenacity and it can be hard to shake your way of thinking about things.
But hopefully you will be able to do that.
He still decided, let's arrange these electron pairs on a cube with the nucleus of the atom supposed to be at the center, and let's keep these electrons, now, nicely collected in pairs. And let's get these pairs as far away from each other as possible, for the same reasons we used to be putting eight electrons as far away from each other as possible, namely, electron repulsion.
Now, the problem of electron repulsion between the pairs was not really solved. And Lewis decided that the pair theory worked so well that he was not going to worry about that, and so we won't worry about it yet, either. You have your four pairs of electrons arranged at the center of four of the edges of the cube, and that geometry is what we call a tetrahedron, which I am drawing the edges of here in pink.
You have a tetrahedral disposition of four pairs of electrons, now, for an atom.
This might again be the neon atom.
But the nice thing about this choice is that if you want to you can make single bonds with this theory, and you can make double bonds -- -- with this theory, like that.
And then, due to the properties of the tetrahedron, you can also make triple bonds. This is marvelous.
You can see that the famous chemist Gilbert Newton Lewis was talking about electronic structure in terms of symmetrical things, like tetrahedra and cubes, as a way of arranging electrons reasonably in space.
This could, again, be our iodine molecule.
This could be, now, our O two molecule.
And, if we continue that progression in the Periodic Table, what is this molecule? Dinitrogen, N two, the major component of our atmosphere, another interesting molecule that we will be talking about as the semester progresses forward. But chemical language and electronic structure theory are very much still permeated with this affection for symmetry and the problems that you can solve using symmetry to analyze electronic structure theory.
Let's talk next about another molecule because we want to talk about Lewis's development of the electron pair theory, but also we want to understand how that relates to acid-base properties. And so, let me draw here another molecule.
And when you look at this molecule, which is the aluminum trichloride molecule, in which I am drawing out explicitly all of the electrons, not forgetting these two.
And having drawn the aluminum trichloride molecule and looked at it like that, you can ask yourself is the number of electrons divisible by eight?
Yes it is. We have a 24 valance electron system here. It is divisible by eight.
Of course, it is also divisible by two.
You can also ask yourself is this molecule, as written, one that satisfies the rule of eight for all four of the atoms? No.
We have a problem there because this thing is electron deficient.
And if you were Lewis, you would want to know, well, if it is electron deficient, this molecule must be doing something about that to get more electrons.
Which atom is missing electrons, here?
The aluminum. How can we give aluminum more electrons, in a structure like this?
Can we get it up to the rule of eight?
Double bonds, awesome.
Beautiful. We can make a double bond.
And, if we do that, we can allow aluminum to satisfy the rule of eight, or allow the rule of eight to satisfy aluminum. Whichever way you want to look at it. And see what we have done, here? We have taken one of the electron pairs, say, this one, and we have moved it in between the aluminum and the chlorine, so it joins the other one in there.
And we get a double bond. And now, everybody has an octet. But what is the problem with that? The problem with that is that chlorine is very electronegative, and it does not want its electrons stolen away by the very electropositive aluminum center.
There are other ways that aluminum can ultimately satisfy its octet, and this is how we get into the realm of "Lewis acid-base theory." And I want to show you one of the other ways that aluminum can satisfy its octet, and then, we are going to move to the computer so we can visualize some of these properties.
If I put my aluminum here -- and do you remember this molecule up here, the ammonia molecule?
I am going to say that if I draw that ammonia molecule in place, here -- The ammonia molecule comes in, and it has its eight electrons.
It is sharing six of them with three hydrogens.
The idea is that it has an extra pair of electrons on that nitrogen in the ammonia molecule, that it can share with the aluminum. And now we go ahead and complete the picture. And we can have eight electrons around everybody.
And so the rule of eight is now satisfied for the three chlorines, for the aluminum, and for the nitrogen of the ammonia molecule. The ammonia molecule is what we call a "Lewis base." And the aluminum trichloride molecule is what we call a "Lewis acid," for reasons that should now be quite clear. And I want to show you how we can look at these things. And if you will give me just a second here, I am going to load the aluminum trichloride molecule up onto the screen.
What we are going to do is remember that there are lots of different ways in which you can visualize molecules.
And I want to show you some of the more interesting ways.
All that is is a picture of the aluminum trichloride molecule with spheres drawn at kind of arbitrary radii.
The size of these spheres is not representative of any particular physical quantity, but we can change that.
And so now, what we are going to do is draw the thing a slightly different way. And what you can see is that the positions of those nuclei are in this flat box.
I made the box kind of flat. It is kind of a slab because this AlCl three molecule is conveniently kind of flat in its structure. And what are we going to do now? We are going to draw a contour surface. And I like this.
We are going to use the solid surface method, first. And can you see that now?
These curves that enclose the four nuclei of the aluminum trichloride molecule do have some physical significance, because what I have done here is taken results, in this case from the quantum chemistry calculation, but you could also take it from an X-ray crystallographic structure determination study.
And I have drawn a three-dimensional contour map.
The value of the electron density at every point on the surface of one of those ovaloid shapes is the same.
Just like when you have a contour map to describe in two dimensions an interesting complicated surface, we can do this in three dimensions as well.
And one of the things you notice, when you look at a surface like this, is that the value of the electron density actually goes down to some low value in between the nuclei, for this.
When you see molecules represented as balls and sticks, or if you represent molecules this way, just showing a pair of dots between the aluminum and chloride, you may not be getting a physically complete picture of the situation.
You can get a much more complete picture if you have access to the actual electron density.
And then, you can even go a little further, and I want to show you something very cool.
First I am going to need to load in a second molecule.
And that will be the product of the first "Lewis acid-base reaction" that we have discussed.
Because, I didn't say it over there, but that was your first exposure to a Lewis acid-base reaction, in which the ammonia molecule comes in and binds to the aluminum trichloride molecule, in order to satisfy the valence requirements of the respective atom types.
And so, here we are going to look at that molecule, and we are going to look at its electron density.
And I think you are going to like this very much.
Here it comes. And then I am going to represent this as a solid surface.
The value of the electron density everywhere on this surface is 0.1 electron density units.
I want you to start thinking about how that shape appears there because I am going to underscore that a little bit more in just a second. But one thing you should notice is that at the NH three molecule, which is pictured at the top of that representation, what you are seeing is that the value of the electron density does not get very small as you go between the nitrogen and hydrogen nuclei because both nitrogen and hydrogen are quite similar in electronegativity as compared with the aluminum atom in this system.
The fascinating thing about illustrating a molecule in this way is that we can actually associate another property with the color on the surface. Now what we are doing is looking at the aluminum trichloride ammonia system.
And let me see if I can make this thing gradually spin, so that I can talk about it without being pinned to my computer. What we have on the surface on each of these electron density isosurface enclosed regions is a color that corresponds with a value of a function that tells us about the propensity of electrons to be paired at that point in space. The Lewis theory is now colored onto the electron density isosurface of this Lewis acid, Lewis base complex molecule. And the values that correspond to those regions in space where electrons are most likely to be found paired up are colored blue.
Where they are least likely to be found paired up are colored kind of red-orange, here.
And then an intermediate color is this green.
And so, you can see that you have electron pairs associated with each of the three NH bonds, up at the top.
You have another electron pair here in blue, which is very proximate to this aluminum center, that is very electron deficient as we had found right over here because it lacks an octet. And then, each of these chlorines is able to attract electron pairs.
Because chlorine is very electronegative, it attracts the electron pairs to itself and sort of withholds them from the aluminum center, but yet, that development of charge separation keeps everything together in what we can call an ionic interaction, an electrostatic interaction.
You can see here covalent bonding, donor-acceptor complex formation, and electrostatic contributions to bonding.
And all of this is just an illustration of the brilliant ideas of Gilbert Newton Lewis.
Free Downloads
Video
- iTunes U (MP4 - 114MB)
- Internet Archive (MP4 - 203MB)
Audio
- iTunes U (MP3 - 12MB)
Subtitle
- English - US (SRT)