Flash and JavaScript are required for this feature.
Download the video from iTunes U or the Internet Archive.
This lecture compresses all the others into one fast video for review of derivatives.
Five of the 6 functions are old, the new one is a STEP function. Slope = DELTA function.
The 6 rules cover f + g, f times g, f divided by g, chains f(g(x)), inverse of f, and then L'HOPITAL for 0/0
The 6 theorems include the Fundamental Theorem of Calculus for INTEGRAL OF DERIVATIVE OF f(x)
Function 1 is f(x) Function 2 is its slope (rate of change) Add up those changes to recover f(x) !!
The MEAN VALUE THEOREM says that if your average speed is 70, then instant speed is 70 at least once
The BINOMIAL THEOREM tells you the series that adds up to the pth power f(x) = (1 + x)^p
Professor Strang's Calculus textbook (1st edition, 1991) is freely available here.
Subtitles are provided through the generous assistance of Jimmy Ren.
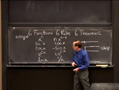
Six Functions, Six Rules, a...
Related Resources
Lecture summary and Practice problems (PDF)
PROFESSOR: Hi. Well, this is sort of a summary lecture for the big group about differential calculus. And it's got a fancy title, Six Functions, that we know. Well, five of them that we know. And a new one-- of course, there has to be something new-- Six Rules, and Six Theorems. So I haven't emphasized theorems, but it seemed like this was an occasion where we could see the main points of the math behind the functions and the rules.
OK. So, here are my first five functions, all familiar. And, what I'm happy about is that, if we understand those five and the rules to create more out of them, we get practically everything, everything we frequently use.
OK. So, I wrote down function one, power of x, and its derivative, function two and its derivative, function three. Function four has, a little bit, something that is important. If it's e to the x, then we know the derivative is e to the x. But, if it's e to the c, x of factor c, comes down. Important case, you could say the chain rule. The derivative is that times the derivative of what's inside, which is the c. And, finally, the natural logarithm with the great derivative of 1/x.
And now, oh, I left space to go from function one backwards, to remember the function that came before it. So, what function has this derivative? I'm looking here at the other generation, the older generation. Well, the function with that derivative is we need the power to be one higher, right? And then, the derivative of that, we need to divide by n plus 1 so that, when we take the derivative, the n plus 1 comes down, cancels this, and gives us x to the n-th. The function that comes before sine x will be-- oh, there was cos x in that direction. In this direction, we need minus cos x because the derivative of minus cos x is plus sine x. But, for this guy, cosine x, that came from sine x.
And, what about this one? What function has this derivative? Well, with exponentials, we expect to see that exponential always, e to the c, x again, but, since this would bring down a c and here we don't want it, we'd better divide by that c. So then, if I take that, that's e to the c, x divided by c, so the c will come down, cancel the c, just the way here. And, oh, we've never figured out log x. That'll be something novel to do for integral calculus. But, I think, if I write down the answer, I think it's x times log x minus x. I believe that works. I would use the product rule on that. x times the derivative of that would be a 1 minus that. And the derivative of that would be a 1, so two ones would cancel, and the product rule would leave me with log x times the derivative of that. It works. It works.
And notice the one beautiful thing in this list, that the case here is great unless I'm dividing by 0. If n is minus 1, I'm in trouble. If n is minus 1, I don't have here something whose-- if n is minus 1, I can't divide by 0. I don't get x to the minus 1 out of x to the 0. That rule fails at n equal minus 1. But look, here, is exactly fills in that whole. Wonderful. Here is the minus 1 power, and here is where it comes from. So that log just filled in the one hole that was left there. OK. Otherwise, you know these guys.
But here's a new one: a step function. A step function, it's 0 and it jumps up to 1 at x equals 0. So, here's x. The function is 0 until it gets to that point. So it's level, then it takes a step up, a jump up, to 1. And let's say it's 1 at that point, so it takes that jump.
All right. OK. That's a function that's actually quite important. And it's sort of like a two-part function, it's got a part to the left and a part to the right. And they don't meet, it's a non-continuous function. Can I figure out what is it that-- so here will be the old generation, what graph do I put there so that the derivative is 0 and then 1? Well, that's not too hard. If I put 0's here, the derivative will be 0. And now, over here, I want the derivative to be a constant 1. And we know that the derivative of x is what I need, so this is 0 and then x, two parts again. And the derivatives of those parts are 0 and then 1. And I often call that a ramp function because it looks a little like a ramp.
OK. What about going this way? Ah, that's a little more interesting because what's the derivative of a step function? What's the slope of a step function? Well, the slope here is certainly 0, and the slope along here is certainly 0, so, is the answer 0? Well, of course not. All the action is at this jump.
And what's the derivative there? Now, a careful person would say there is no derivative there. The limit of delta f/delta x, you don't get a correct answer there because delta f jumps by one, and delta x could be very small. And, as delta x goes to 0, we have 1/0, we have infinite. Well, I say, what? Let's go for infinite. So my derivative is 0 and 0, and, at this point, it's infinite. It's a spike, or sometimes called a delta function. It's 0, and then infinite at one point, and then 0.
And the oddball thing is that the area under that one-point tower, spike, is supposed to be 1. Because, do you remember-- and we'll do more areas if we get to integral calculus-- but, the area under this function is supposed to be this one. The area under the cosine function is sine x. The area under this function should be this one, so the area is 0 here. Run along here. No area under it. Then, I have a one-point spike, and the area is supposed to jump to 1 under that spike, at that single point. That spike is infinitely tall, and it actually has a little area under it.
Ah, well, your teacher may say get that function out of here. That's not a function. And I'm afraid that's a true fact that it's not a real function. So you could say I don't want to see this thing, clear it out. But, actually, that's very useful. It's a model for something that happens very quickly: an instant, an impulse, so I'll leave it there. I'll leave it there, but I'll go on. So, if you don't like it, you don't have to look at it.
OK. So those were the six functions, now for the six rules. Nothing too fancy here. I don't think I really emphasized the most important and simplest rule that, if you have as a combination, like you add two functions, then the derivatives add. Or, if you multiply that function by 2 and that function by 3 before you add, then you multiply the derivatives by 2 and 3 before you add. It's that fact that allowed us-- I mean, you've used it all the time.
If you integrated x plus x squared, you used the sum rule to integrate-- ah, sorry-- took the derivative. If you want the slope of x plus x squared, you would say oh, no problem: 1 plus 2x. 1 coming from the first function, 2x from the x squared function. So the slope of a sum is just the sum of the slopes. You constantly use that to build many more functions out of the simple, anything, x squared plus x cubed plus x 4, if you know its derivative and you're using this rule.
Now, the product rule, we worked through. You've practiced that. The quotient rule is a little messier with this minus sign and the division by g squared. It's a fraction. And then, a little more complicated, was this inverse function. Do you remember that if you start from y equals f of x-- which is what we always have been doing-- and then you say all right, switch it so that x isn't the input anymore, it's now the output, and the input is the y. So you're reversing the function. You're flipping the graph. We did this to get between e to the x and log x. That was the most important case of doing this flip between y equals e to the x and x equals log of y. And the chain rule tells us that the derivative of this inverse function is 1 over the derivative of the original. Nice rule.
And here's the full-scale chain rule. Oh, that deserves to be put inside a box or something because this is a really great way to create new functions as a chain. You start with x. You do g of x, and then that's the input to f. You will know that chain rule. And you remember that that produces a product, the derivative of f times the derivative of g, but there was this little trick, right? This g of x was the y. I'll just remind you that this g of x is the y, and you have to get y out of the answer. Use this to get an answer in terms of x. Wherever you see y, you have to put in g of x. So, that's the chain rule.
And then the final rule that I want to mention is this L'hopital rule about-- well, a lot of calculus is about a ratio of f of x to g of x when it's going to 0/0. What do you do about 0/0? Well, as we're going to some point, like x equals a, if this is going to 0/0, then you're allowed to look. The slopes will tell you how quickly each one is going to 0, and the ratio becomes a ratio of the two slopes.
So, normally then, this answer would be the derivative at a divided by the derivative at a. If we're lucky, this 0/0 thing, when we look at the slopes, isn't 0/0 any more. It's good numbers, and L'hopital gets the answer right. OK. That's a review of L'hopital's rule, just really remembering that that's an important rule that came directly from the idea of the derivative. We're using the important part of the function because the constant term in that function is 0. Good.
OK, are you ready for six theorems? That is a handful, but let's just tackle it. Why not? Why not? OK. So, six functions were easy. Well, we start with the big theorem, the big theorem, the fundamental theorem of calculus. The fundamental theorem of calculus, OK, that ought to be important.
And what does it say? It says that the two operations of going from function one to two by taking the derivative, the slope, the speed, is the reverse of going the other way, from two back to one. It's really saying that, if I start with a function-- Here, this would be one way. If I start with a function, f, I take the derivative to get function two, the speed, the slope. Then, if I go backwards-- which is this integrating that integration symbol that's the core in integral calculus-- if I take the derivative and then take the integral, I'm back to f. And what you actually get in this number is f at-- it depends. It's like a delta f, really. It's the f at the end minus the f at the start.
Maybe you'll remember that. When we talked about it, there was one lecture on big picture of the integral, and there may be more coming, but that was the one where we had this kind of thing. And, in the other direction, if I start with function two, do its integral to get function one, take the derivative of that, then I'm back to function two.
Actually, you're going to say I knew that: function one to two, back to one. Or start with two, go to one, then back to two, that's the fundamental theorem. That those two operations, of taking the derivative, that limit-- You remember what's tricky about all that is that this d,f, d,x, involves a limit as delta x goes to 0. And this integral will also involve a limit as delta x goes to 0. So that's the point at which it became calculus instead of just algebra. Well, important.
I should say, let's assume here, that these functions are all continuous functions. And I'm going to assume that these theorems will apply to continuous functions. And do you remember what that meant? Basically, it meant that that jump function is not continuous. And that delta function is-- well, that's not even a function. The ramp function is continuous but, of course, the derivative isn't.
OK. All right. So, we've got functions that we can draw without raising our pen, without lifting the chalk. And here's the fact about them, that if I have a continuous function on an interval-- so, here is some point, a, and here is some point, b, and my function goes like that. Oh, it doesn't do that. It goes like that. Then this thing says that this maximum is actually reached, and this minimum is actually reached. And any value in-between, anywhere between this height and this height, there are points where the function equals that.
The continuous function hits its maximum, hits its minimum, hits every point in-between. Where, if it wasn't continuous, you see it could go up, and then, suddenly, never reach that point, suddenly drop to there. There's a function not continuous, of course, because it fell down there. And it never reached m because it was this close, as close as it could be. But it never got there because, at the last minute, it jumped down.
OK. So, that's sort of a good theoretical bit about continuous functions. OK. So, that's new. That was not mentioned before. But you can see it by just drawing a picture where it hits the max, hits the min, hits all values in-between. And then, you see the point, y, continuous was needed because, if you let it jump, the result doesn't work.
OK. Here's another thing. This is now called the mean value theorem. That's a neat theorem. OK. Oh. Now, here, our function is going to have a derivative over some region. That function probably had a derivative. OK. OK. So, that function, or this function, f of x, here's the idea of the mean value theorem. This is like delta f/delta x for the whole interval from a to b. Delta x is b minus a, the whole jump. Delta f is f at the end minus f at this end.
So that delta f/delta x is like your average speed over the whole trip. Like you went on the MassPike, right? And you entered at 1:00 o'clock and came out at 4:00 o'clock, so you were on the pike for three hours. And your trip meter shows 200 miles. So your average speed, average speed, was 200 divided by 3, that number of miles per hour. Yeah, about 66 miles-- well, probably illegal. OK. A little over 66 miles an hour: 200/3, so you're slightly over the speed limit.
Well, the mean value theorem catches you because you could say well, but when did I pass the limit? When was I going more than 65? And the mean value theorem says there was a time, there was a moment when your speed, when the speedometer, itself, was exactly. This instant speed equaled the average speed. Shall I say that again? If you travel with a smooth changes of speed, no jumps in speed, then, if I look at the average speed over a delta t, there is some point inside that one where the average speed agrees with the instant speed.
Or you could say, if you prefer slope-- Suppose the average slope, the up over a cross, is 10, So in the time at cross, you eventually got up 10. Then there will be some point when your climbing rate was 10. There'd be some point when that instant slope is also 10. OK. That's the mean value theorem. This is called the mean value. Mean value is another word for average. So the mean value equals the instant value at some point. But we don't know, that point could be anywhere.
OK. Now, I'm ready for the last two theorems. And the first one is called Taylor Series, the Taylor's theorem. And we have touched on that. And what is Taylor Series about? Taylor Series is when you know what's going on at some point x equal a, and you want to know what the function is at some point x near a. So x is near a. And, to a very low approximation, f of x is pretty close to f of a. This is the constant term. That's where the trip started. So this is like a trip meter for a very short trip.
The first thing would be to know what was the trip meter reading at the start. But then the correction term, so this is the calculus term, it's the speed at the start times the time of the trip. If you only keep this, the trip meter isn't moving. When you add on this, you're like following a tangent line. If I try to describe it, you're pretending the speed didn't change. Here, you're pretending the trip meter didn't change. Nothing happened.
Here is the next term. But now, of course, this speed normally changes too. So calculus says there is a term from the second derivative, there's a bending term. This, we would be correct to stop right there on a straight line: constant speed. But now, if the speed is increasing, your trip meter graph is bending upwards, you'd better have a correction from the second derivative. That's the slope of the slope, the rate of change of the rate of change. It's the acceleration.
So, if I had constant acceleration, like I drop this chalk, it accelerates. So, from where I drop it, that gives me its original height. Its original speed might be 0, if I hold onto it. But then, this term would account for the second derivative, the acceleration. And that would give me the right answer, the right answer to the next term, but now I've drawn the famous three dots. So three dots is the way to say there are more terms because the acceleration might not be constant.
What's the next term? If you know the next term, then you and Taylor are square. The next term will be 1/3 factorial, 1/6. It'll be a third derivative of f at the known point times this x minus a cubed. You see that these terms are getting, typically, for a nice function-- and we saw this for e to the x. We saw the Taylor Series for e to the x.
Can I remind you of the Taylor Series for e to the x around the point 0 because e to the x is the greatest function I've spoken about, at all? So, if this was e to the x, it would start out at e to the 0, which is 1. Its slope is 1, so this is 1 times x. Its second derivative is, again, 1. And a is 0 here, so this would be 1/2, 1/2 factorial x squared. And then that next three-dot term would be 1/3 factorial x cube. And you remember what it looks like. So the Taylor Series just looks messy because I'm writing any old f. I'm allowing it to be the start point, to be a, and not necessarily 0. But, typically, it's 0. And the e to the x series is the best example.
But I want to show you one more example. That'll be my last theorem. I just mention it here because it's just like the mean value theorem. If I do stop, suppose I stop here and I don't include the x cube term, the third derivative term, then I've made an error. And, of course, that error depends on what the third derivative is, the one I skipped, the x minus a cube, the thing I skipped, and the 1/3 factorial. And this third derivative is, at some point, between a and x. That's a lot to put in, but the mean value theorem said you could take the derivative at some point in-between, some point along the MassPike. And this is just the same thing, but I'm keeping more terms. I'm quitting at any point, and then I would take the next derivative at somewhere along the MassPike.
What should you learn out of that? I think the idea is Taylor Series. And, of course, we have two possibilities. Either we cut the series off and we make some error, but we get a pretty good answer, or we let the series go forever. And then comes the question. Then we have an infinite number of terms, and then the question is does that series add up to a finite thing like e to the x? Or does it add up to a delta function or something impossible?
So that leads to the question of learning about infinite series. In calculus, Taylor Series is where infinite series come from. And, if we want to go all the way with them, then we have to begin to think about what does it mean for that infinite series to add up to a number, or maybe it just goes off to infinity. Does it converge, or does it diverge? Ah, that would be another lecture or two.
Let me complete today with one more theorem, a famous one, the binomial theorem. So, what's the binomial theorem about? The binomial theorem is about powers of 1 plus x. 1 plus x is a typical binomial: two things, 1 and x. And we have various powers. Well, if the powers are the first power, the second power, the third power, we can write out, we can square 1 plus x, and we can get 1 plus x cubed. And, out of it, we get this. And there would be 1 plus x to the 0-th power. And do you see that there's a whole lot of ones in the neat pattern there? And then there's a 2, and a 3, 3. And if you'd like to know this one, it would be 1, 4, 6, 4, 1 would be the next row of Pascal.
Pascal really had a sense of beauty or art in this triangle of numbers. And that's the triangle you get, Pascal's triangle, if you're taking-- A whole number, a power is 1 plus x to the third power, fourth power, fifth power, sixth power, but what if you're taking to some other power, any power, p? So now I'm interested in this guy to a power of p that, maybe, is not two, three, four, five. It could be 1/2, 1 plus x square root to the 1/2 power, or 1 plus x to the minus 1 power.
All other powers are possible and, for those, the Taylor's theorem. And here's my function. And I could apply Taylor's theorem to find the-- and I'll do it at x equals 0, that's the place Taylor liked the best. So the constant term-- think of this Taylor expansion that we just did-- at x equals 0, this thing is 1. So, the big theory starts out with a 1 for the constant term.
Then what I do for the next term of the Taylor Series? I take the derivative and I put x equals 0. And what do I get then? I get p times x. So this is the constant term: f of 0. This is the derivative: times x minus a divided by 1 factorial. Well, you didn't see all those things because one factorial I didn't write. And then the next term would be the next derivative, of p minus 1 will come down, so you'll have p, p minus 1. You're supposed to divide by 2 factorial. That multiplies x squared.
Well, my point is just that this binomial formula is Taylor's formula. The binomial theorem, with these, this is called a binomial coefficient. Gamblers know all about that, you know? If you've got p things and you want to take two, how many ways to do it? You know, how many ways to get two aces out of a deck, all these things are hidden in those numbers, which gamblers learn or lose.
OK. So, I'll make one last point about the binomial theorem. Those were Taylor Series. This is a Taylor series. What's the difference? The difference is these series stop. This is a series: 1 plus x squared. That's the Taylor Series, but the third derivative is 0, right? The third derivative of that function, because that function's only going up to x squared, the third derivative is 0, so the rest of Taylor Series has died. It's not there. So that's all there is. The derivative of any of those powers, one, two, three, four, five powers, after I take enough derivatives, gone. But, if I take a power like minus 1, or 1/2, or pi, or anything, then I can take derivatives forever without hitting 0.
In other words, this series goes on, and on, and on. Those three dots-- let me move that eraser so you see those three dots-- that signals an infinite series and the question of does it add up to a finite number, what's going on with infinite series? But, for the moment, my point is just this is what calculus can do. If you not only take that slope, but the slope of the slope, and the third derivative, and all higher derivatives, that's what Taylor Series tells you. OK. So that's the, in some way, high point of the highlights of calculus, and I sure hope they're helpful to you. Thank you.
FEMALE VOICE: This has been a production of MIT OpenCourseWare and Gilbert Strang. Funding for this video was provided by the Lord Foundation. To help OCW continue to provide free and open access to MIT courses, please make a donation at ocw.mit.edu/donate.
Free Downloads
Video
- iTunes U (MP4 - 84MB)
- Internet Archive (MP4 - 84MB)
Subtitle
- English - US (SRT)