Flash and JavaScript are required for this feature.
Download the video from iTunes U or the Internet Archive.
Topics covered: Regulation of Productivity
Instructor: Prof. Penny Chisholm
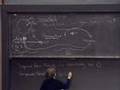
19: Regulation of Productivity
Related Resources
Handout (PDF)
So, today we're going to talk about, we've talked about primary productivity on a global scale last time. And today, we're going to talk about what regulates that productivity. In other words, last time we just talked about on average the amount of carbon and biomass that was distributed among the ecosystems of the globe. And we talked about deficiencies of transfer of that biomass through food webs, etc. By the way, the one universal thing that everybody seems to like are the DVDs at the end of the class, which is good. Unfortunately, in today's class we are not going to have one. So you have something to look forward to on Wednesday. I'll show you one at the end that is really one of the cooler ones of the collection. It has nothing to do with the lecture but I'm going to show it to you anyway. OK, so today we are going to talk about what regulates the productivity. We've talked about these complex systems in ecology, and the feedback mechanisms. So, in the context of productivity we're going to talk about the factors, the abiotic factors, to the non-living parts of the Earth, that regulate this productivity and how the productivity feeds back on those. And then Wednesday, we're going to put it all together in an analysis of global biogeochemical cycles, how the elements in the globe cycle, and how that's mediated by organisms. And then, that will be the end of the segment. And when I come back, we're going to move on to population and community ecology, which will feel like a totally different subject to you. So, we'll talk more about organisms. And some of you said it was so interesting to see math last time in the last lecture even if they were just efficiencies. Well, when you get to population ecology, you're going to have real math and you'll actually have differential equations. So, if you like that you have something to look forward to. If you don't like it, well, it's not too bad. OK, so today the lecture will be in two halves. We'll talk about terrestrial productivity, and then aquatic productivity and what regulates it. And, can you see in the back or do I need to turn, I'm going to have to turn the lights down, never mind. So, because we have some colored images, so let's start with terrestrial productivity. And look at this map, oops, wrong ones. I think that might be enough to see it, which is a satellite image. You've seen several of these so far. So just showing the gradients of productivity on a global scale, where we're just looking at the land now. Where it's green, you have high levels of productivity. These are grams carbon per meter squared per year. Yellow is intermediate, and red is very low levels of productivity. So, what determines this distribution of productivity in terrestrial ecosystems? Well, got any ideas? This is not hard. What's a key factor in regulating plant growth on land? Light, absolutely. That's a given. But looking at this map, what's probably more important? Water, exactly. If we plot on a global scale, if we go around to all these ecosystems, and we look at the average annual rainfall and we plot it against net primary productivity, you all know what NPP is right, net primary productivity, you get a graph that look something like this. Each one of these dots would be an ecosystem. And this one is millimeters rain per year. So, despite the Sahara Desert, and this might be a tropical rain forest. And they scatter, but there's some sort of general relationship like that, increasing NPP with increasing rainfall. Well, what else is probably important? What else is different between the Sahara Desert and the northern US? Temperature. Exactly, which is not a good example for comparison. Let's take tropical rain forests and the northern US. How's that? But you get something like this. In other words, it doesn't always map directly onto temperature alone. But on average, you find that places with higher temperature have higher productivity if there's adequate water. So, does a relationship, there's an interaction between the water and the temperature. So, those are the key factors for terrestrial ecosystems. Now, what we have, so there's light, nutrients, I mean light, rain, and temperature, rainfall. What about nutrients? Anybody who's had plants and their room, or nurtured our garden knows that nutrients are very important. You have to fertilize in order to get the most growth. So, how does that work? Well, now we are going to do an analysis of a terrestrial ecosystem. We're going to use a tree. Some ecosystems you have, that's a rock in case you didn't recognize it. And we have soil. These are components. And, we've talked about this over, and over, and over now. Photosynthesis is the key, taking up CO2, evolving oxygen, we are going to call this biosynthesis. That's the mass from gas. So, that's CO2 plus, and now we're going to add something. And these are not balanced chemical reactions, OK? These are just to give you an idea of what's going on. So, CO2 plus, there's all the elements required for life, OK? In other words, for a plant to grow, it doesn't only need CO2 and water. It needs all of these elements required for life. And they are converted to organic forms of those elements. And then oxygen is evolved. So, we are in a general sense, just modifying the equation for photosynthesis to include all of the elements that are required for life. So that's the biosynthesis. And then, the tree, these are leaves in case you didn't recognize them, that are falling to the soil. And the leaves fall down to the soil and they become, what? What's that organic manner? You learn it last time. It's the whale falling to the bottom of the ocean, and if that was a carcass, the general term for dead, organic matter is called detritus. And there is a detritivore food web, remember? And we had some discussion with students after class whether we were detritivores, because in a sense we are because we eat dead meat, right? We don't eat live meat. It doesn't matter. You don't have to know that. Forget that. But it's interesting to think about. So, the leaves fall down to the soil. They are acted upon by the heterotrophic bacteria, the bacteria that use organic carbon. And, what happens is that those bacteria and fungi, and worms, and everything that chews on organic matter are responsible for regenerating these elements in the soil. So, we are going to call that regeneration. And that's basically, simply the back reaction of this, OK? So, you are starting with organic carbon. And, organic carbon, organic PNS, and it's converting it back to the inorganic forms so that they're available for the tree to take up again. So, this is the cycle of biosynthesis regeneration, and then take it up again. Some of the feedback I got on the lectures when people said that they found it interesting to think about how everything in nature is recycled and used. Now, in some ecosystems, there's another form of available nutrients: calcium here, you have cations, potassium from rocks, magnesium. All of these are also in this equation. When I go dot, dot, dot, dot, it's every element that's required for life. And in some ecosystems, the [disillusion?] of these elements from rocks is an important renewal route for nutrients in the ecosystem, OK? So these are the two, basically in terrestrial ecosystems, there is the rock source, and there's the regeneration source. And it turns out that different terrestrial ecosystems have different relative dependence on these two sources. And this is an interesting phenomena. So, tropical rain forests, like in the Amazon, these are the forests that we're concerned about losing for many reasons. And these have, essentially, no number one up here. Tropical rain forests have essentially no renewal nutrients from bedrock. And temperate forests, however, have a combination of one and two. They can have renewal. The bedrock is exposed, and the water cycle helps dissolve the rock, and renews the nutrients to the system. So, if we look at the soil to biomass ratio of phosphorus and nitrogen in the temperate forests versus the tropical, we see something like this. In the tropical rain forests, all of the nutrients in the system are basically tied up in the biomass. And it's highly dependent, then, on this regeneration cycle. The trees fall down, it's regenerated, it's taken up right away from the soil whereas in the temperate system, you see the opposite where there's a much higher proportion of nutrients in the soil relative to the tropical system. And what that means is that if you cut down a tropical rain forest, which they're doing, converting to farmland, you will only get a few years of productivity out of that farmland because once you shut down the forests and haul away the trees, you've hauled away most of the nutrients in that ecosystem that are available to fuel productivity. And they can't be renewed from bedrock because they don't have bedrock there. So, that's one of the tragedies of cutting down these forests when they probably would have more economic value by harvesting some of the natural products from the forests. OK, so when we get to aquatic productivity, we are going to see that we have these same biosynthesis and regeneration processes. So, that's what we're going to move to now. And let's look at the distribution of aquatic productivity. That's too much. A while. Can you see that in the back OK, the colors? All right. So, now we're just looking at the ocean's ecosystem. And areas that are blue and green are less productive than the areas that are red and yellow in the system. So you can see all of the coastal regions; we have coastal upwelling that we're going to talk about a lot with nutrients fueling that, that is very important. And on the whole north Atlantic here we'll talk about that. But before we talk about what regulates aquatic productivity, now I am going to turn the lights out, I thought I'd give you a tour. Since you all know trees look like but you don't know what primary producers in the ocean ecosystems look like, I'm going to give you a quick tour through the phytoplankton, which as you know, are my favorite organisms. So, the aquatic productivity is dominated by these microscopic plants. There are over 20, 00 species, but we really have no idea how many there are. Wherever there's water, they exist. They range from 0.5 to 1,000 µ in diameter. And as I told you in the first lecture, there's as much genetic information and a liter of seawater that contains these primary producers and all the bacteria but they live with than there is in the human genome. So here's some of my favorites. These are marine diatoms. This is a silicon shell. This is a single cell. It's about 30 µ in diameter. And this is made out of amorphous silicon. It's essentially opal. And here's another one. They come in different shapes and sizes. They're just really incredibly beautiful. And people are just starting to study what mechanisms are responsible for laying down these exquisite architectures. Here's another one. To me this always remind me of the Coliseum for some reason. These are pillbox shaped cells. And they have two halves like that. And when they grow, when they divide, one half lays down another half inside of it. Now, what's going to happen ultimately if these are rigid? They get smaller. One lineage gets smaller, and smaller, and smaller. Well, both of them get smaller, and smaller. And this group of organisms has this really neat system where when it gets really tiny, they differentiate into egg and sperm. They made, and that they make a giant cell again. And they start the whole thing. It's cool. Just to show you some of the things people have studied, people have wondered why have they evolved this very heavy armor? And of course, the first thing you think about is resistance to predation. But there was never any evidence for that. And so, I just found this recent study in nature that shows where they actually measured. I thought MIT students look like this because they actually measure the force that it takes to crush one of these cells. Here's the study. There is a diatom [thrustule? and they're putting a measured force on it to see what would crush it. And they were able to show that the amount of force that it takes is enough to have a selective advantage against the crunching parts of the zooplankton that eat them. I also want to point out, this is from the website of Dr. Angela Belcher, who is a professor here at MIT and material sciences. And she is studying diatoms. Here's a diatom. She's studying them as a material, this amorphous silicon, looking at the way it's laid down. And she's also studying coccolithophores, which is another group of my favorite organisms. They have these calcium carbonate plates. Again, this is a single cell. But it's cell wall is made up of calcium carbonate plates. And they come in all different shapes and sizes. Here's a really weird one with these huge, they're called coccoliths. And these cells, this is a satellite image of reflection, of light. In these cells, the calcium carbonate, reflects light. And this is a coccolithophore bloom somewhere I think in the Bering Sea. And we can measure these, but we have no idea what causes a particular species to bloom at a particular point in time. It's one of the challenges of oceanography. Here's another group of organisms. The cyanobacteria: we've talked about them a little bit. These are prokaryotic cells that can fix nitrogen. They're one of the few groups of microbes that can take nitrogen gas from the atmosphere and convert it to ammonia, which draws it in to the food web. So you can actually see a bloom here. That one's called trichodesmium. It grows out in the open Atlantic and Pacific. Here's a bloom of trichodesmium sucking nitrogen into the ecosystem, converting it into ammonia, making it available to the other organisms. Here's the organism we work on, unfortunately very boring looking, not as exciting looking at the other ones. And now, this is just under a light microscope. These are less than a micron in diameter. But if you shine blue light on them, they fluoresce red. The chlorophyll in them fluoresces red. And these are the smallest and simplest photosynthetic cell. They have 1,700 genes. And with that, I call them the essence of life because with 1, 00 genes, they can convert CO2, nitrogen, phosphorus, all inorganic compounds, basically this rock over here, in sunlight into life. And this happens to be like that dominates the oceans. They are the most abundant cell in the oceans. In some areas, it's about 50% of the total chlorophyll. So, they are basically a lean, mean photosynthesis machine, and we're trying to understand everything about it. OK, does a digression, hopefully not a diversion. So, what regulates aquatic primary productivity? Have to turn the lights back on. Before we get into that totally, I want to draw a typical, what we call a water column in an aquatic ecosystem. And I have seen this little red, sticky thing here. This is from last year, and it says they don't understand these axes. See, I remember from year to year. So, if you don't understand something and doing, because I can tell when students come up afterwards that I've completely lost you. First of all, oceanographers plot things upside down. So this is depth. So, depth goes down, which makes sense, right? And then, whatever we are plotting against depth is on this axis. So, in this graph I'm going to make, we are going to plot net primary productivity, temperature, nutrients, a bunch of different variables. OK, and for oceans, this is about, well, we'll just say 2,000 m, and for lakes, say, 200 m for a deep lake. So, I'm drawing here sort of a generic picture of a water column in either a lake or ocean. OK, so do we have colored chalk? Not here, OK. Sometimes it used to float around. Well, you're going to have to use your imagination. So first of all, let's plot light as a function of depth. What does that look like? Like that, exactly. It's going to decay exponentially. In lakes, this is about 10 m, and in oceans this is about 100 m. Oh, and this was a question somebody gave in the instant feedback. Somebody said, I find it hard to believe that all life in the oceans disappears where there is no more light. And, you're absolutely right. It's only the photosynthetic life that disappears when there's no more light. There's lots of life below there that is using organic carbon. So, if you're here, that the answer to your question. OK, so then, now we're going to plot temperature which looks something like this. And, this is what's called the [thermocline?]. And we can also think of this as density. Because colder water is more dense than warmer water, right, you must have learned that, did you learn that somewhere? Did you learn that somewhere? Where did you learn that? Fourth grade, great, well-prepared. So, up on the surface we have biosynthesis here where there's light, and so it's exactly the same reaction as we have over here. And now it gets to the terrestrial ecosystem. The production you have in the surface water is you have phytoplankton photosynthesizing, making organic matter. They're being eaten by zooplankton, by fish that are making feces, etc., that are being eaten by the detritivores. But the net effect of this teeming food web they saw in the DVD last time, is that there's going to be organic carbon that falls down below this lit zone. OK, and that was the whale calling out to make, no, not purposefully, but telling down and making carbon available to the food web in the deep water. So down here, you have regeneration. So this is light. And this is in the dark in the system. But it's directly analogous to the biosynthesis and regeneration system there. So the other thing we want to plot on this is nutrients. They are drawn down to very low levels in the surface water in the lit layer because the phytoplankton are sucking them up. They are nutrient limited. They're sucking up the nitrogen, phosphorus, et cetera. And then, as the carbon and all of that range down to the deep water, it's regenerated, and then the nutrients are regenerated. So that's why you see the gradient of the low nutrients here and high nutrients here, because the bacteria in the deep water are breaking down the carbon and releasing them. OK, so if we look at this map, we can see that for aquatic ecosystems, obviously water is not limiting. So water is an important regulator. Light is a very important regulator of productivity down to about in this region. And nutrients, it turns out, are very important. And it's nutrients that really determine the tapestry of this map that we're looking at. And what I'm going to do for the rest of the class is explain it lakes and oceans how the physical forces make these nutrients available in certain regions more than in other regions and explain this. OK, so first let's look at lake ecosystems. So, what we're showing here is a year in the life of a temperate lake. So this might be the Mystic Lakes out in Arlington or something like that. Well, maybe that doesn't freeze over, I don't know. But anyway, a lake that freezes over in the winter. So let's start during the summer, and here's this basic graph showing the thermocline, the nutrient depletion in the surface, and this one indicates that you actually have oxygen depletion in the deep water because of all of this organic matter from productivity raining down and being consumed by heterotrophic organisms that consume oxygen. So, as fall comes, and this is the important part, in the summertime, this layer is mixed. So, it's isothermal. In the fall, you have the winds and the surface cools. And as this density gradient here starts to break down, do the cooling in the winds. And so, you have this mixing. It's called fall overturn, which [then trains?] these nutrients from the deep water into the surface. So that's what way you get the nutrients for the deep water back up into the surface, whereas in the summertime, the gradient is maintained because of this density barrier, and the mixing can't bring this down. And then in the winter, you have the ice cover that obviously everything then is just isothermal. There's not much going on, but there is some photosynthesis. And then in the spring, the surface waters start to warm up, the ice melts, you have overturn, and brings the water up from the deep water. In the ocean, in lakes, this can mix all the way to the bottom, OK? In the oceans, there's no force of nature that can mix all the way down to 2, 00 m. So you have this thermocline in the oceans, but it's in a relatively small fraction of the total water column. So the scale here is way off. We're going from 100 m down to 2, 00. So in the ocean, it's just this tiny little, all this action in the surface. So we need another mechanism. We can't mix all the way down to the deep ocean. So, we need another mechanism for bringing nutrients to the surface. And we're going to talk about, there's four different ways. There's four different ways that the deep water nutrients are brought back up where there is light, because you have to have liked for photosynthesis to use the nutrients. And one is episodic mixing. I'm just going to list them, and then we're going to go through them: coastal upwelling -- -- equatorial upwelling, and on much longer time scales, what's called the ìoceanic conveyor beltî in quotes, which is basically local ocean circulation. So let's go through these. In the oceans, episodic mixing, let's go back. Now, just pretend this is an ocean, and that this goes down to 2, 00 m, and there's a thermocline. What happens in the oceans is that you just have little, episodic mixing events that you wrote a great here to get little bursts of nutrients injected into the lit area. That's seasonal mixing, but it never mixes all the way to the bottom. And we can see, I'll show you where, this right here is the north Atlantic's bloom. And in the springtime, you see major bloom there due to this episodic mixing in the North Atlantic, which have high winds and a lot of mixing. OK, so there are also ocean currents caused by this coastal upwelling phenomenon, especially along the western coasts of continents. And I don't have time to go into this. You need a whole course and physical oceanography to really understand this because it has to do with the whole global ocean circulation that causes this upwelling along the coasts. But, I'm going to show you how this works in this movie, or a little movie. I guess that's as dark as we're going to get. This is a cross-section of a coastal ocean. So, here's the coastline. Here's the surface of the ocean. And these little molecules here are CO2. Can you see blue? This is probably not going to work because of this filming. Well, we'll see what happens. OK, so going to go through it in a still, and then I'll show the movie. But what you're going to see is as blue patch upwelling along the coast here, in CO2 molecules are coming up with it. OK, here's the blue. That's nutrients, nitrogen, phosphorus, etc. The wind is blowing offshore causing the surface waters to move in that direction. They have to be replaced by something, so we're bringing the deep water up to replace that moving surface water. And as that comes up, the CO2 comes up and is released. And then you have a phytoplankton bloom from the nutrients, and then the CO2 sucked back in again and you have oxygen going out. And, here it goes, the movie. Upwelling: The Movie. There comes the CO2. Here are the nutrients. CO2 out, CO2 back in. These are phytoplankton, this green blobs. That's a bloom. So you have now the phytoplankton falling, big bloom, organic carbon going down and being regenerated. So, it's a very dynamic system and that's why you have a lot of high-intensity fisheries along the coasts, especially the western coast of continents because of this upwelling; there's lots of nutrients, lots of phytoplankton, lots of fish. A dramatic example of the power of the surface currents, and how they affect upwelling is this phenomenon called El NiÒo. I think I'll skip the slide. You don't have it in hand out, anyway. But here's an animation showing the changes in the productivity in the Pacific Ocean along the equator. Here's an El NiÒo. And I'll explain how that works in a minute. Here's a normal year where you have these phytoplankton blooms, and let's look at it in this. You've seen this one before, but let's look at it more closely. There's the equatorial bloom caused by upwelling along the equator and are normally year. And as we go around, this is like three years in the life of the globe. See, there is high productivity at the Amazon where the Amazon's empty. Now, we are going to zoom in here, and this is a normal year. And that's an El NiÒo year. You see, there's very little productivity, and is very little upwelling along the coasts here. And that El NiÒo in Spanish, what does it mean? It refers to the Christ Child because this happens around Christmastime roughly every seven years. And what happens in a phenomenon is it turned out that people have studied enough for years. It's a global phenomenon in which the prevailing currents in the whole Pacific Ocean shift from going in this direction which causes the upwelling here to going in this direction, which brings warm water suppressing the upwelling and reducing the nutrient input into the system. OK, so here's an El NiÒo year, and directly compared to non-El NiÒo year. And that's all due to physical force is changing the nutrient delivery. So here's equatorial upwelling, and then finally on a global scale, over very long periods of time, you can imagine that these upwelling events would not be enough to bring, you have this constant rain of organic matter coming from the surface waters. And this nutrient reservoir in the deep waters, none of these upwelling events are enough to bring all that back and renew the system. So you need a bigger force than that on a global scale over long time periods. And that's what's called the great oceanic conveyor belt. And this is really important, because I think people think of the oceans as a static, understandably, you look out there; it looks like a bunch of water with the surface waters and with the deep waters. And if you throw something in the deep waters it's going to stay there and you don't have to worry about it anymore. A lot of people want to bury nuclear waste in the deep water. And the point is that that's not true. The oceans are all interconnected, and if I'm a water molecule, the average amount of time, and I'm traveling with the currents, over a thousand years, I will make this whole journey where I go along the surface waters and then I get to the North Atlantic, and because the waters are cooled and there's very high winds in the North Atlantic, you have cold water and of the high winds cause high evaporation. So you have saltier water, so, cold, saltier water up here sinks. And that actually is a force that drives this whole global circulation. And so, I'm cruising along here. I get here, and I sink. And then I go for this long journey down the bottom. And of course, it's much more complicated. This is grossly oversimplified. But I go this long journey to the bottom of the Atlantic in these deep ocean currents, and then somewhere along the line, I get brought up again through zones of upwelling here, or maybe I would meander up here and get brought up again. [I'm just one atom on average? . So, through these global ocean currents, the deep water eventually comes up to the surface, bringing those nutrients back. Where it comes in contact with the light and the phytoplankton, and they photosynthesize and they take up the nutrients and they make organic carbonate, and it all settles down again to the bottom. So, it's a cycle. And if you didn't have that, the thing would run down. If you didn't have the deep water coming up eventually coming up somewhere, the system would just run down and you do have a big anoxic bottom of the ocean. And who knows what would happen? So, this is really important. OK, so finally, I've talked about nutrients just in general, what nutrients are the most important? And, it turns out that there are some nutrients that are in much less supply that are required by the plants. And this is what's called, so, what nutrients are important? Now, of course, they're all important but some are more important in regulation than others. And there's something called the law of the minimum. And it states that the growth of a plant will be limited -- -- by that element that is in least supply relative, this is the important part, to the requirements of the plant or the phytoplankton. When I say plant, it could be phytoplankton or a tree, or a plant, or whatever. And this is the important part. So how we figure out what the requirements for elements are above plants? You might grab it, harvested, grind it up, and measure the ratio of the elements in that plant. So, for example, if you do that, for most plants you get something on the order of, at least for most phytoplankton, which are my preferred plant, you get the ratio of carbon, nitrogen, and phosphorus, of 106 atoms of carbon [for? 16 nitrogen per one of phosphorus. So this tells you in what ratio they need these elements in order to grow. So then you look in the environment and you ask, what are the ratios available? So, say if the water has a ratio of, what's going to be the most living element in that system for that plant? Exactly, nitrogen. And, alternatively, you could have something like this. And what would be limiting there? Phosphorus limits. And it turns out that in most aquatic ecosystems, for now we're going to say that nitrogen and phosphorus are the important limiting factors. And just to show you, again, that ecologists do experiments, here's an experimental lakes area in Ontario, where there are 22 different lakes set aside for research. And in this particular set of lakes, this is a control lake, and this is the experimental lake. They added phosphorus to the lake. And you can see the phytoplankton bloom by only adding phosphorus. They didn't add anything else. And that means that phosphorus was in least supply relative to the other nutrients. And the interesting thing that happened here was that when they added the phosphorus, that makes phosphorus in great abundance relative to nitrogen. And what that did is make nitrogen the limiting factor. And when nitrogen is the limiting factor, what organisms might have an advantage? We talked about them. Yeah, there you go, nitrogen fixing organisms. So, if nitrogen is limiting, only organisms that can take it from the atmosphere can get more nitrogen than the other organisms. So they are favored. And what happens is you fertilize with phosphorus. You get blooms of nitrogen fixing organisms. It's really an interesting phenomenon. But nitrogen and phosphorus are, one or the other is limiting in lakes. And in large areas of the oceans, nitrogen and phosphorus are also limiting, except we've learned recently that there are areas of the oceans where iron is actually a limiting factor. And this was an experiment that was done by oceanographers. And there is Alaska just to get you oriented. This is the North Pacific where they went out with a boat and they made it a patch. They added iron just to a patch of ocean. And I can tell you about this. We were involved in some of these experiments. It started out with a 10 km x 10 km patch and showed that if you add iron you get a bloom of phytoplankton. And this is a satellite image of that phytoplankton bloom. And in the last lecture, I'm going to tell you all about those iron fertilization experiments, and the implications for how we are going to use the oceans in the future. So, take-home messages: we'll talk about that next time. You can take them home, but I don't want to keep you over.
Free Downloads
Video
- iTunes U (MP4 - 106MB)
- Internet Archive (MP4 - 192MB)
Subtitle
- English - US (SRT)