Flash and JavaScript are required for this feature.
Download the video from iTunes U or the Internet Archive.
Topics covered: Introduction
Instructor: Prof. Graham Walker
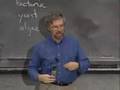
1: Introduction
I am a professor in the Biology Department at MIT. And I will be co-teaching this course with Penny Chisholm who is a professor in the Department of Civil and Environmental Engineering, as well as a professor in the Biology Department. As well, Penny was recently featured in the journal Nature. She is a very well-known oceanographer who has become deservedly famous for discovering a very small bacterium that's capable of carrying out photosynthesis. For many years oceanographers used filters whose holes were big enough that this bacterium went through. And so when they were doing their studies of the ocean and how the biomass that was there and all the fluxes and so on, they didn't know this organism existed which can form up to 50% of the biomass in parts of the ocean. So Penny is really a wonderful lecturer, a wonderful person, and she'll be teaching the component of 7.014 which deals with ecology and the environment. And this is a section of 7. 14 that makes it different from the other two versions, 7.012 and 7.013. So for me personally this is an absolutely wonderful and exciting opportunity to be able to teach this Introductory Biology course. For some of you I know that biology is going to either be a major part of your career or quite possibly, even if you're in engineering or something else, you will find yourselves working with a biological system. You can see that happening all over campus these days that more and more engineering departments are finding that they're working on problems that come from biology or have a biological component. I'm sure there are at least a few of you here who are only here because it's a required course and you might well wish you were somewhere else. However, I will do my best to try to communicate to you why you need to know some biology, too. I think most of you know you can hardly pick up a newspaper these days without running into something that demands a knowledge of biology, something about stem cells, something about cloning humans, something about a new drug, lots of things having to do with biological affects on the environment and so on. You're also going to be confronted with decisions about your health, about the health of your loved ones concerning cancer, concerning whether a child might have birth defects, all sort of issues that will affect your personal lives that demand an understanding of biology. So I feel with some passion that whether you think you're going to need biology in your professional career or not, everyone in this institution needs some understanding of biology to just live their ordinary lives. I also think as MIT students you're going to be looked at, as you go through your lives, as people who are knowledgeable about science and engineering. And you'll be asked questions that go far beyond your immediate area of expertise. And again I think that's another reason for needing to know some of biology. Anyway, it's an absolutely wonderful time to teach biology because things have just been exploding over the last two or three decades and things are moving faster than ever. And another wonderful thing about teaching biology is that MIT is an absolutely marvelous place to teach it. Just to sort of drive this home, in the Biology Department alone there are four Nobel laureates who got honored for critical discoveries in biology. Gobin Khorana who is just down the hall from me synthesized the first gene. It was an extraordinary feat of synthesis of organic chemistry synthesizing DNA. When I was an undergrad I was a chemistry major but I had to take an introductory biology course. And at that point the DNA wasn't mentioned in the high school course. So the first time that I heard about DNA was in my introductory biology course. And that determined by career direction. I thought that was such an interesting molecule that I wanted to work on it and I talked myself into one of the labs in Ottawa, Canada where I grew up that was trying to synthesize DNA, synthesize pieces of the gene. And, as it turned out later, competing unsuccessfully with my now colleague Gobin Khorana. Susumu Tonegawa got a Nobel prize for discovering the amazing molecular operations that underlie the diversity of the immune system. Your immune system has the capacity to recognize viruses and bacteria, all sorts of different pathogens, including molecules. It can recognize molecules that haven't even ever been synthesized in the history of life. And we'll talk towards the end of the course about the way that happens. And you'll see why Susumu got his Nobel prize. Phil Sharp got his for discovering RNA splicing completely unanticipated component of the very heart of molecular biology. And then Bob Horvitz who was of pure mind when he started at MIT at the same time and our labs were side by side for many years, got his Nobel prize in 2002 for discovering a phenomenon, the general term is ìprogrammedî cell death. And it plays all sorts of important roles in biology from sculpting the shapes of organs and tissues. We initially have webs when we're developing between our fingers, and those go away because of the programmed cell death that the cells that were making the web disappear. And another role of that is prevent cancer. That if cells sense that something is very messed up they have a sort of suicide program that would make them destroy themselves. And if that doesn't happen those cells could go on and become more and more abnormal and eventually turn into an invasive cancer. Anyway, there's a picture of Bob when the institute was celebrating his Nobel prize. He was getting a congratulatory kiss from Martha Constantine-Paton, also a professor in the Biology Department who happens to be Bob's wife. Most of you know that the human genome has now been sequenced. That was one of the huge undertakings and most important undertakings in modern biology over the last while. It was an incredible feat. Each cell has, as most of you know, 46 chromosomes. And that's a total of about two meters of DNA in every human cell. And that two meters of DNA is composed of about 3 million DNA base pairs, these letters A, T, G and C that we'll be talking about as we go through the course. So to sequence the genome you had to work out the sequence of the exact run of these A, G, T and Cs along the backbone for 3 billion base pairs. And somewhere that genome encodes somewhere between 20,000 and 30,000 genes. And we'll be talking about the proteins that are encoded by most of those genes and their important roles as we go on in the course. What some of you may not know is the key role that MIT played in this. About a third of the genome was sequenced at the Whitehead MIT Genome Center. And here are some of the robots that were used to sequence that DNA. And that sequencing effort was led by Eric Lander whose name some of you may recognize because he teaches the fall version of 7. 12 along with Bob Weinberg. So here are just a few examples of why I think it's important for you to understand biology regardless of whether you're going to go on and use it professionally. Most of you know one of the biggest challenges we face on this planet is this AIDS epidemic. It's caused by a certain kind of virus called HIV-1 that gets into particular cells of your immune system that normally defends us against infection and destroys those. And then people die from infections by other organisms that normally you can fight off. It's a huge problem with vast societal and economic implications. And it's one that we're still, as a mankind still trying to deal with and grapple with. Here's another example. Just a couple of years ago there was the scar of anthrax. It's a bacterium that's very pathogenic and kills its host fairly easily. It does it by making particular toxins. The details shown here don't matter, but just reminding you that this is something that was in the front pages of the paper just a little while ago. A couple of years ago, when I was teaching this, we had the scare of the SARS virus that went all the way during the course when I was teaching it from the initial discovery of the virus to the actual sequencing of the genome which had happened by later on in this course. Smallpox was a disease we thought that we eliminated, but now it's come back as a bioterrorism treat and there is increased study of smallpox. It's something we have to worry about again. Here's another example. This is a picture showing the start of a transgenic animal. We'll talk about how this process goes later in the course. And it's related also to this whole issue of cloning, using the sense of the word such as in trying to clone a human or clone an animal to make a genetically identical copy. So you'll see there are a couple of different uses of the word cloning as we go through the course. There's a lot of fuss in the news and the newspapers about genetically modified food, and people have different positions on it. Here's a case where I think the benefit of a genetically modified food could hardly be argued with. About two-thirds of the world's population uses rice as their primary source of food. One of the problems with rice is that it doesn't make beta-carotene. And beta carotene is what our bodies take and use to make vitamin A. And if you have a vitamin A deficiency people are prone to infection. They have immune deficiencies in their immune system and blindness. And this deficiency of vitamin A caused by eating rice afflicts about 400 million people worldwide. Well, rice is only two chemical steps away from being able to make beta carotene. This is a genetically modified version of rice that has those two extra steps inserted in it. And you can see it's golden because it's making beta carotene. If people were to eat that form of rice then they wouldn't have this problem with vitamin A deficiency. Penny Chisholm in her part of the course will be considering things that are more global level. Here's a picture of our planet, and there are issues that you know about there. This is the carbon dioxide levels in the atmosphere rising. And because of the emission of fluorocarbons in the environment And that's a real phenomenon. This shows from 1960 to 1995. damage our cells if we were exposed to it. absorbs UV, a critical component of ultraviolet radiation that would This is associated with a global warming that again is undeniable this large hole developed over Antarctic. If that had spread, got a Nobel prize for that. Ozone is important because it that it's happening. You'll see stuff in the papers. Right here at MIT Mario Molina in EAPS discovered the ozone hole and And these gases, particularly carbon dioxide and methane, And Penny will talk to you a little more about that. it would have been a real disaster. And that seems to be, the efforts they're known as greenhouse gases are playing a role in that. gases and, hence, in global warming. And probably mankind is playing a role in the production of those to cut down the release of fluorocarbons seem to be helping with that. But, again, Penny will have more to say. Those of you who've lived around here for a little bit probably know that the fishing industry just locally has had a very hard time. There is a fishing boat just up in Gloucester, just up the coast less than an hour from here. And part of the problems, again, are caused by mismanagement of the resources where the fish have been, stocks have been over-fished so some of the fisheries have come to the point of collapse or near. Places such as the Grand Banks off of Newfoundland there has been a collapse, and it's not at all clear that it can be reversed and whether they'll be cod in large quantities every again there. OK. So there a variety of ways that we can study biology. And I think I'm going to begin by outlining how we do that. Biology is an experimental science and it's one of the really important themes that we'll run through in this course. You cannot study biology by just sitting down with a pen and paper in a room and thinking. You have to get out and find out what's there. You need to make observations. You need to try experiments. You need to formulate hypotheses and test them and either modify your hypothesis or reject it and so on. But it's a continual cycle of experimentation and making hypotheses and testing. It's the Scientific Method at work. But you can carry that out at different levels. The very highest level would be the biosphere. Here's one example. That's earth. It's here. There are many, many species of life that are on there. There are many more than a million. And the estimates of how many there are range from, in total, 10 to the 20 million estimated. And one of the big worries right at the moment is that as rain forests are being depleted, parts of the world that are a rich source of biological diversity, as those are disappearing we're losing diversity at quite a rate. Just a couple of years ago I had a chance to fly over a part of Brazil. And it was just scary to see from the plane how the rain forest was just being cut down. And you could just see how fast some of the rain forest was disappearing, and with it many different types of species that only can live there. One can look instead, going down a level, at an ecosystem which is a particular environment -- -- and the species of life found in it. And just to give you a couple of examples of that, it could be a salt marsh as shown here. Or here's an interesting environment that Penny Chisholm will tell you more about. This is a black smoker. There's a vent several miles deep in the Pacific Ocean. The water temperature that's gushing out of here is around 360 degree centigrade. And there's a particular community of life that's able to grow around these deep vents. And Penny will tell you more about that. Then going down yet another level you can come to a population. And this is interacting -- -- or interbreeding organisms. An example might be the fiddler crabs in a salt marsh. Or here we see an interesting population. These are tube worms that are up to a meter or more in length that you find down at these black smokers. If we move down yet another level -- -- we come to organisms. Organisms have three important sorts of characteristics that we'll talk quite a bit about in this course. They carry out metabolism which is the sum of all the different chemical reactions necessary for life. They undergo regulated growth -- -- and they reproduce. And the sort of fundamental unit of life that we will talk about over and over again in this course is known as a cell. And life comes in two kinds of species. There is unicellular life where the organism is just a single cell and multicellular forms of life that are made of many different types of cells. What's a cell? One of the secrets to life. It's a little tiny bit of the universe that's surrounded by a boundary. And it's given the special name of a membrane. It's selective, not very permeable to most things. And cells are able to put little importers and exporters and things that control the passage of things across the membrane by isolating the inside of a cell from all the rest of the universe. That is one of the principles that makes life possible. We have a couple of examples of organisms here. Here are some clams that grow down at those black smokers, and they can get pretty large. And, as I say, Penny will talk a bit more about this. And we have this really amazing diversity of life forms that we find on this planet. However, if we think about this division into unicellular and multicellular organisms. Unicellular organisms include things that you're familiar with. They're bacteria. There is a picture of just E. coli cells. And we'll be talking about E. coli quite a bit as a model organism as we go through the course. By studying E. coli, scientists have learned many important things that apply to all of life. Another kind of important single-celled organism, unicellular organism is yeast. Those are pictures of yeast saccharomyces that are used in baking bread or in brewing beer or making wine. And another one you're all familiar with are algae which are single-celled organisms that are able to carry out photosynthesis. And we'll be talking about that. If we think of an example of a multicellular organism then we see there are different levels at which we can think about this. We could take, for example, a picture of me, just an anatomically correct diagram here, that I'm made up, as you are, of about ten to the fourteenth human cells. We all started out as a fertilized egg, which is a single cell. And by the time we're grown up, where we have about ten to the fourteenth human cells. Just a tremendous amount of cell growth that had to happen and specialization. The other thing you may not appreciate is that we have an ecosystem inside us in our gastrointestinal tract. This part, the intestine having the highest concentration of microorganisms. But there are about ten to the fourteenth bacteria also inside of our gut. So we're actually almost the same number of human cells and bacterial cells. And if we don't have those bacteria then our digestive systems don't work well. So if we go down from a whole organism, a whole multicellular organism, a level, then we come to an organ. An example of that might be an eye. And I think we have a diagram of an eye which is made up of different parts. If we go down another level we come to a tissue. Which is now you can begin to see that tissue are made up of groups of specialized cells. An example might be the retina of an eye. And if we continue to go downwards we'll get to single cells. And at this point we're at the same level of the tail as when we're talking about a unicellular organism. If we continue down then -- -- we can get to organelles. These are involved in energy production, energy management. And mitochondrion and chloroplasts are the two principle examples of organelles that we'll talk about. And if we go down yet another level of organization we get to molecules. And part of the reason that biology has flourished so well over the last few decades at MIT is there has been a real emphasis on looking at things at a cellular and molecular level. So you're going to be hearing a lot about cells and a lot about molecules as we go through this course. Here's an example of rhodopsin. That's a protein. We'll be talking about what proteins are, but it's a very important class of molecule in nature. In this case, proteins involved in sensing light and play an important part in your vision. Here is another protein. You cannot really tell what it's doing by just looking at it, but in this case this is one of the lethal factors that is made by anthrax. It's one of the proteins that anthrax makes that's capable of killing you if you get infected with it. Here's another molecule we'll talk about in great detail. This is DNA. You probably all know it's a double helix, two strands of DNA that are held together by forces we'll be discussing. It's an absolutely beautiful molecule. It's fascinated me through all of my life. And we'll be talking quite a bit about that as the course goes on. OK. So if we're thinking about cells there are two important kinds of cells that one finds on this planet. Prokaryotic cells. Prokaryotic organisms and eukaryotic organisms. They each are made of cells that are distinguishable from each other. I've indicated that a cell is a little bit of the universe that's surrounded by a boundary or a membrane. But inside there, inside of this is the DNA which functions as the genetic material. It's the blueprint for everything that that cell is going to make and be able to do. Ultimately everything is encoded there. And in a prokaryotic cell the DNA is free within this membrane. The eukaryotic cell also has a membrane. But the DNA inside is inside another membrane compartment known as the nucleus. And this is the DNA. These prokaryotic cells tend to be of the order of a kilometer in length. And eukaryotic cells are usually larger, can be ten to a hundred kilometers. There's quite a bit of variation, but that gives you at least some sense of the range. Now, I've for years, when I did a diagram like this, I wanted to somehow be able to show you that these cells were impressive than just what's on the board. So here are a couple of pictures to try and do that. This shows a picture of E. coli swimming along. And the way this image is being taken lets you see what are called flagella but which are basically the propellers that E. coli have that let it swim through the water. These are long structures made of proteins that are several times the body length of the bacterium. And there's a molecular motor imbedded in the bacterium that whirls it around at about somewhere between 10,000 and 100, 00 RPM. And that's what drives the bacteria forward. So that was a prokaryotic cell. Here's a paramecium. This is a single-celled eukaryotic organism. And, as you can see here, it's capable of movement as well. In this case it has cilia along the outside that allow it to move. Here's an interesting one. I don't know if any of you can guess what these were. These were cells from the skin of a mouse. They're on an Auger surface. And, as you can see, they too can move. There are a couple of things that are important about this. I got this slide from Linda Griffith who is in the Biological Engineering Department. At the time I got it from her I think it was in Chemical Engineering several years ago. And what was important about this, apart from it being a very nice little movie showing you a mammalian cell moving around, was that I saw Linda show this during one of her research seminars. So here is an engineer at MIT who was showing this picture as part of her research talk. And I think those of you who are going onto engineering, you may be surprised at the extent to which you need to know about biology as you go through your professional careers. OK. So one of the great discoveries that has happened over the last few years that came out of our ability to look at DNA and RNA was the discovery that the forms of life that are prokaryotic actually split into two distinct Kingdoms that are very, very different. The archaea and the bacteria. And just to give you a sense of the diversity of life, I'll just mention a couple of these. These archaea look like bacteria but they are diverged from the bacteria as they are from the eukaryotes. So there were sort of three really major Kingdoms of Life. And the archaea, many of them can live in specialized environments. For example, sulfolobus can live at about 90 degrees centigrade and a pH of somewhere between 1 and 2. So if you see something like a hot springs, there are organisms such as this that are able to grow in that environment. Or there are halophyes, salt-loving archaea that can grow, for example, in formula sodium chloride. And if you've, for example, ever flown into San Francisco airport coming up from the south over San Jose, you've seen things that look sort of like these pictures where seawater is being evaporated down to collect the salt. And you'll see they're colored, and the reason they're colored is that these halobacteria are photobacteria that are able to use light as an energy source. And they make pigments that absorb the light, and that's why these salt areas get colored. A third example would be methanogens. These are organisms that produce methane. If you've walked into a lake and stepped on the bottom and seen little bubbles come up, those are little bubbles of methane. Or another place where you find methanogens are inside of cows. Now, some of you may not know that the cow is more or less a walking anaerobic fermentor here. If we have an anatomically correct picture of a cow. The inside of the cow, there's a large chamber known as the rumen where there's no oxygen, and there's a culture of microorganisms there that include methanogens. And it's this combination of microorganisms that enables cows to each grass that we cannot manage to get energy from. And as a byproduct of this specialized type of metabolism produces methane. And a cow burps something of the order of 400 liters a day of methane. OK. So one last thing then just to kind of pull this all together is that these organelles that I mentioned, which are also membrane compartments that are found in eukaryotic cells, are the mitochondria -- coli or streptococcus that causes strep throat or the lactic acid you're more familiar with. Things like E. bacteria that causes the milk to turn into yogurt which some of you probably had for lunch today. That these organelles, the mitochondria and the chloroplast were derived from particular type of this point that these arose from bacteria that were things that bacteria that probably first got transiently associated with developing eukaryotic cells sometime back in evolution, and eventually became captured and became a permanent part of the eukaryotic cell. The mitochondrion is thought to have derived from something that looks like today's present day -- or chloroplast. There's pretty strong evidence at rizobia, which we'll talk about, that form an intracellular infection of plants, or rickettsia which is chronic intracellular pathogen. The mitochondrion look as though they came from something related to that. The chloroplasts look as though they came from a bacterium that was able to carry on photosynthesis which we'll also be talking about. I want to close by giving you just a quick little snapshot of evolution because I'm hoping this will maybe make some of the things that we talk about in this course clearer. So what we're going to do is we're going to look back from 4. billion years ago when the earth was just forming -- -- to now. I'm just going to try and give you a few key sort of landmarks as we go along. So about 4.5 billion years ago there was methane, carbon dioxide, ammonium, hydrogen gas, nitrogen gas, water, but importantly no oxygen at that point. There was a lot of debate as to how life initially came. One of the prevalent theories at this point is there's something called an RNA world. This is just a hypothesis in which it's thought that perhaps the molecule RNA, which we'll talk about, played role as both something that was able to catalyze chemical reactions and therefore did things actively and also stored information. But, in any case, the best guess is that the first life that was about 3. billion years ago, somewhere in that vicinity. It was something that probably resembled most closely a present-day bacterium, a single-celled organism, something like that. Now, initially when life got started it's thought that there were a lot of organic chemicals that had been made as a consequence of lightening strikes and all sorts of chemistry that had happened so there was sort of a soup of some kind, some molecules that could be used. So probably these first organisms where able to basically use some preformed nutrients. And then as the soup began to get depleted by using it they had to learn to synthesize, at least develop systems that would synthesize these building blocks. And they also had to begin to worry about what to use as energy. And so somewhere in here, something that I'll call, in a silly way, photosynthesis released number one. But this was a system that enabled the organism to capture energy from sunlight so that it wasn't now dependent on getting energy by eating some preformed ingredient. It was then able to take carbon dioxide and make it into forms that were useful, of carbon that were useful for life, and it produced molecules such as sulfur as a waste product. There was a bit later in evolution, somewhere in here, something we might think of as photosynthesis release two. This was an improved version of photosynthesis. It captured more energy, worked better, but it developed, it had a waste product which was oxygen. Well, oxygen hadn't been in our atmosphere. And the first thing that sort of happened was that the world started to rust. All the iron, a lot of the iron started to interact with the oxygen. And Penny will tell you that at the base of the sea there are huge beds of iron oxide that came from this slow rusting of the earth. And so it took many, many years before oxygen levels started to rise. As you know it's about 20% of our atmosphere now. Even at this stage it was only a few percent of our, made up a few percent of our atmosphere, even by here in evolution. The first eukaryotic cell is thought to have appeared somewhere here. Again, it was likely a single-celled organism like some of those pictures I showed you. And evolution continued to go. Somewhere around a billion years ago sex was evolved which enabled eukaryotic organisms to exchange genetic material, and therefore evolve at a fast rate than they could previously. The Cambrian Period was about a 0. billion to 0.6 billion years ago. And there was a veritable explosion of life forms. And you can still see in the fossil records how much diversity was generated at that point, some of which went on to become life forms and other which probably were more evolutionary dead ends. Finally we get to the dinosaurs that were about 245 to 65 million years ago which would place them somewhere here on this timeline. So in honor of this course, I've commissioned a full scale model of anatomically correct [NOISE OBSCURES]. So we'll put our dinosaur here, if I can get him to stay put for a minute. All right. And at this point in evolution things started to get interesting. So somewhere about here, 4 million years ago we've got the first evidence of hominoids. Maybe 20,000 years ago we found the cave paintings in France. And then there was the Roman Empire and Columbus discovered America. And you were born and the Red Sox won the World Series and the Patriots have just won the Super Bowl. And we are now here at the peak of evolution which is, as you all know, the MIT student. So we'll put our MIT student here, who I can probably not get to stay put because you can never get MIT students to stay anywhere. But, in any case, this is sort of a silly demonstration. But there is a very profound reason why I'm doing it. And I must say I don't think I'd ever fully appreciated it until I actually thought of doing this demo for the class. But what I think you can see is that evolution, for the most part, happened at the single cell level. Many people tended to think evolution, that was about dinosaurs and all that stuff. We can say that dinosaurs are, practically now, most of evolution occurred at the level of single cells, and that all this amazing diversity we see around us was very recent embellishments in evolution. So that means when you study biology at the cellular and molecular level you find tremendous commonalities. If you look inside a sulfolobus growing in hot spring, if you look inside an E. coli, if you look inside a yeast and you look inside one of our cells you find that, to a huge extent, many, many of the cellular components are common. They arose similarly in evolution that they're shared by all forms of life. Of course, there are some things that developed later and are different. But that's one of the reasons that you can learn so much by studying biology at the cellular molecular level and why we'll emphasize it a fair bit in this course. The other thing that I'd like to make out of this, a theme that you'll hear along is that organisms modify their environment. You can see that in the case of oxygen back when the earth formed. There was no oxygen in our atmosphere. Now we have a lot of it. The reason it's there is because it was generated by organisms carrying out photosynthesis and generating oxygen as a waste product. And that was an absolutely critical thing to enable creatures such as ourselves, which are dependent on oxygen for us just to be alive, if we hadn't had this change in environment things like us could have, organisms like us couldn't have evolved. So, anyway, I hope that will give you a little sort of snapshot of evolution and will help guide your understanding of this course. We'll see you at the next lecture.
Free Downloads
Video
- iTunes U (MP4 - 105MB)
- Internet Archive (MP4 - 184MB)
Subtitle
- English - US (SRT)