Flash and JavaScript are required for this feature.
Download the video from iTunes U or the Internet Archive.
Description: Students give presentations on their projects: photovoltaic lights for Lighting Africa, NextGen modules, and NextGen performance simulation.
This video is from the Fall 2011 version of the class.
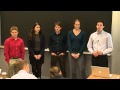
2011 Student Project Presen...
The following content is provided under a Creative Commons license. Your support will help MIT OpenCourseWare continue to offer high quality educational resources for free. To make a donation or view additional materials from hundreds of MIT courses, visit MIT OpenCourseWare at ocw.mit.edu.
STUDENT 1: We would like to present to you PV Next Gen, Earth-Abundant Materials. So the reason we're doing this project is because there are current limits to the high-quality crystalline silicon cells that we're producing. There's too high of an energy cost and too high of a carbon footprint.
So we decided to identify likely sources of failure for earth-abundant PV. We focus on tin sulfide, lead sulfide, and cuprous oxide. So we took the approach of subjecting these PV cells into a climate chamber to sort of simulate aging, and accelerated aging, and the effects of long-term operation out in the field.
So for a little bit of a background, this is a graph that we'd like to provide you with. If you notice, the graph is centered around zero, which is crystalline silicon. And on the x-axis, it's kind of inverted. So the negative's on the right.
But this graph sort of illustrates that where we want to be, in our research, is in that quadrant within the circle. Because that's where we have the lowest materials extraction costs. And we have the highest annual electricity potential. So those are the kind of technologies that we want to be looking at. And as you can see in the arrowhead, cuprous oxide and lead sulfide are in that quadrant.
JOEL: So we've established, we've showed you how these kinds of materials can be less expensive and, potentially, produce more electricity than silicon. However, they haven't had the same kinds of decades of experience in testing as silicon has. So we're trying to figure out how they could possibly fail under actual years of operation in the field.
First, we're going to talk about tin sulfide. Certainly, tin and sulfur are both elements with which we're very familiar. Tin has a wide manufacturing base. Sulfur is part of all of our biology, pretty easy to find.
The band gap of this material is 1.3 electronvolts, a little bit higher than silicon. But that, actually, brings it closer to the optimum of the Shockley-Quiesser efficiency limits for a single-junction solar cell. The maximum possible efficiency is 32%.
However, the current record efficiency, for tin sulfide cells, is only 1.3%. So that gives you an idea of the state of development of this field. Often, it seems that devices are limited by their charge extraction.
There is an interface between the absorber layer and buffer layers that block electron and hole transport. I'm going to talk about that a little more on the next slide. But this interface has been, unfortunately, not very well characterized to date and is often a site of high recombination rates that can limit the current extracted.
However, tin sulfide is a good material for photovoltaics. Because it's crystal structure is amenable to doping. You can add substituent atoms and tune the electronic properties that way.
Also, it has a high absorption coefficient. So it can be made into thin film devices. And the device that we're going to show you today was made using atomic layer deposition.
Here's that schematic that I was mentioning. So as in this diagram, the light is coming in from the bottom. Don't be alarmed.
The bottom substrate, that things are subsequently deposited onto, is a glass pane that is coated with fluorine-doped tin oxide. This is like indium tin oxide. It's another transparent conducting material.
And then, this layer here is the tin sulfide absorber. And you can see that on either side of it, there is a hole-blocking layer and an electron-blocking layer, which prevents charge from flowing in the opposite direction that we want it to. And then, both the absorber and the front contact have these metal stack electrodes patterned onto them, onto the same side of gold, copper, and molybdenum.
So this is a manufacturing idea that could make tabbing easier and eliminate shading losses. Because all of your electronics contact to one side on the back here. However, as we'll discuss later, it comes with some challenges of its own.
JOLENE: The other material that we investigated, for this project, was lead sulfide. Lead sulfide is also an earth-abundant material. Lead is even more abundant than tin.
The bulk band gap of lead sulfide is only 0.41 eV, which is a little bit too low to make it a good observer material for photovoltaics. But if you make nanocrystals, you can tune the band gap. So there's confinement effects that make it so that the band gap can actually become larger.
So we looked at lead sulfide quantum dot solar cells. And in particular, we looked at lead sulfide quantum dots and a PCBM, which is a fullerene derivative heterojunction, where the lead sulfide is a p-type material. The PCBM is the n-type material. And we just made a bilayer heterojunction.
With these materials, the major potential failure mode, that we identified, was oxidation of both the lead sulfide quantum dots and the fullerenes. And then, there's also possibilities of hydrolysis of this PCBM esther group upon reaction with the EVA encapsulant material. That was something that we thought might be a problem.
The current record efficiency for these lead sulfide quantum dot solar cells is only 2.1%. Also something, more work needs to be done in improving that efficiency. But a major advantage of this material system is that both the lead sulfide quantum dots and the fullerenes can be solution-processed. So they can be spin cast onto your substrate, which makes the processing cost very low, especially compared to growing a single crystalline wafer.
The device architecture that we used is, again, glass coated with a transparent conducting oxide electrode. And then, there's just a bilayer heterojunction with the two observing materials. And then, finally, it's a metal contact on the opposite side.
For our case, I've shown the band structure of the devices that we used. And, in particular, I wanted to point out that the top electrode, in our case, was actually aluminum because of the band alignment. The work function of aluminum makes it so that the top band aligns well with the PCBM.
And you can get charge extraction the way that you want. And, again, our devices, the quantum dots were tuned so that the band gap was about 1 eV. And these are the parameters for the devices that we used.
STUDENT 4: All right, so what we did is our initial plan was to collect IV curves of before and after encapsulation of our solar cells, and after a period of time spent in the environmental chamber. The encapsulation that we chose, from a variety of materials, was EVA. Because it's an industry standard.
For environmental chamber, we kept it at 85 degrees Celsius and at 85% relative humidity to accelerate the degradation process. Then, we took photos of our cells over a period of seven days. Here's a schematic of an encapsulated cell. It's a layer of glass followed by a layer of EVA, then the solar cell, another layer of EVA, and then a TPE backsheet-- again industry standard.
For our tin sulfide, what we did is we actually omitted the glass layer in our encapsulation. Because the cell came with glass, was made with glass. So to make the contact, so we did is we applied silver paste to attach the tabbing. And we baked them at 45 degrees C to evaporate the solvent.
This yielded very delicate contacts. So we had to encapsulate immediately in order to hold the contacts in place. Unfortunately, as Joel was mentioning about how the two electrodes are on the back, during the encapsulation process, we shorted our cell. So we were unable to take an IV curve.
For the lead sulfide, initially-- as Jolene said-- we had aluminum contacts. Unfortunately, when exposed to air, this forms a layer of aluminum oxide, which is nearly impossible to solder to. So we switched the aluminum with silver, even though it's less than ideal for a charge extraction.
And with these contacts, we were only able to achieve a contact with a large amount of solder. And again, we could not encapsulate. Because we risk breaking the cell with these contacts.
What we decided to do, then, was encapsulate unsoldered cells with aluminum contacts, and do a qualitative assessment of the color change, which would indicate a reaction with the encapsulant or with the water oxygen. So basically, unfortunately, despite our initial plan, we were unable to collect IV curves for either type of cell after encapsulation.
DANNY: So we did a series of cross-sectional [INAUDIBLE] images on tin sulfide device before and after several days in the environmental chamber. So on the left, you have an image of the tin sulfide layer before exposure to the chamber. And we can see the columnar structure of the tin sulfide layer.
However, after several days, on the right is an image of the tin sulfide layer having some changes, having undergone some changes to its structure. This may be due to either thermal degradation or a result of [? cleavage ?] of the cell. We also did a series of photos over the course of several days on the devices.
And we noticed that there was some color changes in the device. The tin sulfide layer has undergone, the color of tin sulfide layer darkened over time, especially near the corners of the cell, like around this edge here. For the photo on the extreme right, you may notice some yellowish tinge. And that is due to the lighting the photo was taken in and not an actual change in the cell.
So as for lead sulfide, we did a similar series of photos on the device. And you notice a similar color change has occurred across the device. You also notice that at the four corners, there are contacts there. And these contacts have been tarnished significantly. So we think that the color changes, on both devices, may be due to, one, the leakage of the encapsulant, two, the reaction with the encapsulant, and lastly, due to thermal degradation.
KATHERINE: So if we're going to achieve terawatt-scale electricity output with solar energy, we need new materials that are cheap and efficient. However, the materials we investigated still have a lot of work to be done before they can achieve that. In particular, they have very low efficiencies.
And that's something that absolutely needs to be overcome. However, we focused more on some of the other issues, such as the contacts. Right now, they're still in the research phase. So, for example, using aluminum as a contact is fine.
However, when moving into commercialization, aluminum contacts will have to be replaced with something else. Or something else will have to be deposited on top of the aluminum. We saw degradation of the encapsulated cells, which indicates that the current encapsulant will not work with these new materials.
In particular, we think that we need an encapsulant that will, does not have an acidic degradation product. Because that was a serious issue with the PbS quantum dot solar cells, with the fullerene part can react with acid and degrade. So we think, we did talk about wanting class Evb?
JOEL: PVB.
KATHERINE: PVB. But we did not have access to that material. And we think that the method that we came up with of testing materials in the environmental chamber will be useful in the future. And ideally, you could test different encapsulants and see how well the IV curves stay after the environmental testing.
So we'd like to thank several people for helping with our project, in particular Andy at Fraunhofer, who's actually here today. We had to go to Fraunhofer several times to-- we had several iterations of getting the cells. And then, once we put them in the chamber, we had to take pictures every day. So he was there and was able to help us with that.
Also we'd like to thank Darcy of the Bawendi Group for making the PbS quantum dot cells for us. And also the Gordon Group for making the tin sulfide cells. And then, finally Professor Buonassisi and Joe for teaching us all the fundamentals of the devices.
So before we go to questions, we'd like to pass around the cells we had. While people are asking questions, we ask that you don't take them out of the bag or the boxes just because some them contain lead, which it can be harmful. But we can pass this around while we open for questions.
PROFESSOR: Thank you, group,
[APPLAUSE]
JOEL: David.
AUDIENCE: So why, does FTO have a different work function from ITO? Or for what was the reason that you used FTO in the consultant itself?
JOEL: I'd like to direct that question to Danny.
DANNY: FTO is typically much cheaper compared to ITO. So for research purposes, we prefer FTO.
JOEL: Is it, light leaves out the indium, right?
DANNY: Right. So indium's a rare matel. And it's very expensive and the price is going up. So we try not to use indium in our TCO.
AUDIENCE: Is it the same conductivity?
DANNY: No. For ITO, the conductivity is much better but, yeah, much better than FTO.
JOEL: Still working on the transparent oxide side of earth-abundancy. Different project, though, I guess.
AUDIENCE: Can you guys go back to the environmental chamber pictures for, I think, tin sulfide? Yeah. So, yeah, can you just walk me through this again? The yellow is not what my eye should be drawn to?
JOEL: Yeah, that's a really unfortunate side effect of the way that the camera was that day. But it's, I think, it would show up better if we turn down the room lights. But there is a darkening from Day 2 to Day 3, and then, also, to Day 6. But that's kind of overwhelmed by the yellow glare. Yeah, maybe now you can see it a little bit better.
And we also wanted to point out that although this happens across the whole device, there's particularly more change around the edges. So that to us says that there's definitely something happening here where the atmosphere is leaking in through the encapsulant and reacting with the device. Because that will happen from the outside in, as opposed to a reaction with the encapsulant itself or a thermal process, which would affect the whole device uniformly.
AUDIENCE: And this was EVA?
JOEL: Yes.
AUDIENCE: So, I mean, EVA's a pretty standard encapsulant material.
JOEL: Yep.
AUDIENCE: Do you think that there is something like the EVA process that you used? Or do you think there's some reaction between the tin sulfide and the EVA that caused these EVA films to not be good encapsulants?
JOLENE: So this one, the process that we used to encapsulate these were not at Fraunhofer. These were encapsulated in Joel's lab. And so I think that, and the devices, both devices that we worked with were kind of hard.
They aren't meant to be encapsulated. So I think that part of the problem is just the geometry of the device and the presses that we used and everything. We probably just didn't get a very good seal around the outside.
And so in this case in particular, I think that it's more likely that it's just not a very good seal. So we're getting some leakage rather than the tin sulfide is reacting with the EVA. For the quantum dots, it's a little bit less clear if there's a reaction with the EVA or not.
Because there's a little bit, there's both degradation from, or color changes from the outside that affect particularly the outside. And there's, also, color changes that affect the whole device. And so, then, it's either the quantum dots are somehow changing or there may be some kind of reaction with the encapsulant. But when we took the SEM images, the EDX aspect of it was not working. So it was difficult to do any kind of elemental analysis there.
AUDIENCE: And just one other question, in this sort of accelerated aging process, how long would you want to see stability for a, say, a 30-year or 20-year? If you were going to warranty this cell for me, how long would you might say?
KATHERINE: I think, usually, they do at least six weeks.
AUDIENCE: Six weeks?
JOEL: Yeah.
JOLENE: Yeah.
KATHERINE: And so this was, we didn't have six weeks. But we still [INAUDIBLE] that said. So.
JOLENE: More work should be done.
AUDIENCE: So how long's your warranty on the cell?
JOLENE: Like a day.
[LAUGHTER]
JOEL: There were other encapsulants available at Fraunhofer. Notably, there's this, a thermoplastic and an ionomer encapsulant. But these are both new and have entirely proprietary chemical composition. So we didn't think it would be very informative to study for our project.
Because if we saw something happen, we wouldn't really be able to hypothesize where that came from. But there are other materials out there besides the standard EVA and the polyvinyl butyrate, the PVB that Katherine mentioned at the end. Just not quite as far along. Yeah.
AUDIENCE: So how would you compare the degradation between ITO and FTO like--
JOEL: The degradation of ITO and FTO?
AUDIENCE: How would you compare this? Which one would you pick [INAUDIBLE]? I mean, in terms of cost, OK. [INAUDIBLE]. In terms of technique, it would be--
AUDIENCE: So can you repeat the question?
JOEL: The question is if there's a difference in the degradation between ITO, the indium tin oxide, and FTO, the fluorine doped tin oxide that we used in our tin sulfide cells. Yeah. Do you know anything about that, Danny?
DANNY: Yeah, so they normally degrade when you expose them to extended temperatures above 650 degrees Celsius, which is unlikely to happen in a normal day situation. So there's no difference in this [INAUDIBLE].
Yeah, we didn't expose them to 650 Celsius in our accelerated testing either.
AUDIENCE: I have a question about the aluminum contacts. First of all, how did you deposit them?
JOLENE: The aluminum contacts were thermally evaporated onto the, yeah.
AUDIENCE: So would it be possible just to evaporate the [INAUDIBLE].
JOLENE: Yeah, absolutely, absolutely. We were working with cells that had previously been made. That this was not our group, we were getting materials from, generous donations from another group.
And so there were cells that had already been made, already had aluminum contacts on them. And so then, it would've been much more involved to get other people in other groups to do stuff for us. So we just used the materials that we had.
AUDIENCE: And I'm guessing they don't see these problems because they don't solder their contacts, just scrub them?
JOLENE: Yeah. All the tests for the lead sulfide cells are done in a glovebox, an inner atmosphere. And they have pins that just come down.
AUDIENCE: Right, so it just [INAUDIBLE].
JOLENE: Yeah. Mm-hm?
PROFESSOR: Ashley.
AUDIENCE: I'm just curious, more of a logistical question, did you guys have a camera in the environmental chamber? Or do you have to take them out each day?
KATHERINE: And so we would have to turn off the chamber, cool it down, take the cell out, take a picture, put the cell back in, then turn it back on.
AUDIENCE: OK.
JOEL: So in a sense, we actually put extra stress on them. Because there was thermal cycling [INAUDIBLE].
AUDIENCE: So maybe a two-day warranty [INAUDIBLE].
KATHERINE: Mm-hm.
AUDIENCE: Did you try leaving the cells [INAUDIBLE] in the glovebox after you [INAUDIBLE] go down and, then, try to [INAUDIBLE]?
AUDIENCE: Can he just repeat the question again, too? Sorry.
KATHERINE: Yeah, so he was asking about the contacting, the PVS devices. And we did have, we had two things we did. One was we took them in the glovebox and tried to scratch off the aluminum oxide layer, and then put silver paste on, and then make the contacts to that. And that still didn't work.
And then, we also got new devices with silver contacts on them. And that also didn't work. Because it either would stick to the device, or it would stick to the contact. But it wouldn't stick to both at once. And they would just peel off.
JOLENE: The contacts on those devices are less than 50 nanometers thick. So it, in general, soldering to them was going to be challenging.
STUDENT 1: We tried hard.
[LAUGHTER]
JOLENE: Yep.
AUDIENCE: Is there any future plans to continue with this work, with either Bawendi or Gordon Group? Or are they, was just kind of included?
JOLENE: Well, I think both of these materials are so much still in the trying to boost efficiency phase that it is less important to be able to say, to talk about the long-term stability than to say that they're even viable materials to begin with. I think that they are interested in the results. But, yeah.
JOEL: I mean, this is Danny's thesis, so.
[LAUGHTER]
DANNY: All right, so [INAUDIBLE] from Gordon's Group, we have much more problems much greater than whether they can last a week or two.
[LAUGHTER]
PROFESSOR: One more question, guys. Yes.
AUDIENCE: Do you IV curves for Day 1 and Day 8?
KATHERINE: Yeah, so we weren't able to get IV curves. Because the tin sulfide self-shorted, and we weren't able to make contacts to the lead sulfide, so.
AUDIENCE: Make enough as to make up for what would be the drop in-- [INAUDIBLE].
KATHERINE: So Danny has taken tin sulfide IV curves before the encapsulation, right?
DANNY: Right. So I [INAUDIBLE] encapsulation and they do what you see here, pretty decent IV curve. But before we did the encapsulation, the cell shorted and [INAUDIBLE] died. So we couldn't-- there's no point in doing IV curve after the test.
KATHERINE: So we took them. But it's just a straight line.
AUDIENCE: [INAUDIBLE]
JOEL: All right, Thanks, guys. Let's thank our speakers again.
[APPLAUSE]
JACKSON: All right, this is Ron, Rachel, Ben, Kirsten. And I'm Jackson. I'm going to introduce the Lighting Africa project. So the main problem behind our project is the following. There's about 580 million people living off-the-grid in Africa, and about 10 million small businesses operating off-grid in Africa.
And as you can imagine, after dark, they have a major lighting problem. So kids need lighting for education, for reading, for studying, et cetera. And then, families need it to interact with one another. And small businesses, a lot of them would like to continue operating after dark.
And currently, what they do is they use kerosene. But there's many problems with kerosene lamps. So the first of which is that it's a major health issue.
So burning kerosene is about, and like, for one night, is about smoking two packs of cigarettes in terms of health issues. In addition, it's very expensive for these families. They can barely afford to eat. And so buying a weekly supply of kerosene is extremely hard for them.
And furthermore, actually the widespread use of kerosene, although low per capita, is causing a large increase in CO2 in the atmosphere. So this brings up a large opportunity for us. And the World Bank Lighting Africa project has proposed the following solution.
And that's solar portable lanterns, or SPLs. And these are small devices, maybe this big, that have a compact fluorescent or an LED on them. And they have solar panels on top.
And you set the whole device out during the day. And you can bring it in during the night and use it to light your homes. And these are, right now, of a fairly small market share, compared to the kerosene, but are growing very substantially with a 40% to 50% projected annual growth. And as you can see from the graph, I'm sure you guys are aware Africa has a huge solar resource as a great opportunity.
So the goals for our specific project are the following. How will next generation solar technologies help bring solar PV lights to rural sub-Saharan Africa? So our specific project is the following. How we looked at the next generation of solar, so future technologies that are emerging in the next six months to 10 years, and how they're going to specifically affect our project.
So to do this, we looked at a few different time frames. We looked at the six to 12 month time frame, the one to five year time frame, and the five to 10 year time frame, and made technological prospectus of each one, looked at a wide gamut of different technologies, and selected a few that we thought would most positively affect our project. And we focus on the one to five year time frame. Because we thought that this would be the most important to look at.
So to do this, we looked at a few, a couple different consumers. So the first consumer is a low cost consumer. And this is somebody who can barely afford to eat every day and, really, buys kerosene, maybe, on a week-to-week basis. It's extremely expensive for them.
So we need to minimize the costs of these devices as much as possible so that they have a low buyback time with compared to kerosene. And these devices are often integrated. So there's a solar panel on the device. And you put the whole device out during the day. And you bring the whole device in at night.
The second consumer we looked at is a higher performance device. And so this is a consumer who, maybe, has a little bit more money although still very poor relative to our standards. And then, often these devices are separated.
So you'll have a separate solar panel that goes out during the day. And then, you'll bring either the solar panel in or leave it outside. And maybe there's a cable that connects it. And sometimes, these are even on the roof. And these have a little bit some added features that I'll talk about in a little bit.
So to look at this, we looked at a few different metrics. So the first, obviously, is cost. And that's the most important metric. So we looked at dollars per watt of all these different devices, projected dollars per watt.
And like I said before, minimizing cost is the most important thing. Because these people need an attractive device that will pay itself back fairly quickly in terms of kerosene, and will be affordable. The second thing we looked at is a performance, so efficiency, basically.
So we looked at the parameter watts per centimeter squared. And so we need to minimize the size so that it can fit on a device if it's integrated, or minimize the size of a solar panel if you're going to lug it in and out every day. And as performance goes up, you can get a few added features.
So one of the, actually, big problems that, maybe, is surprising is there's about 50 million off-grid mobile phones at the current time. So these people live off-grid. They need a place to charge their mobile phones. And often, they have to bring in to the city center, or they use hand cranks or something to charge their mobile phones.
And it's expensive and difficult for them to charge. So adding a charging feature, to these devices, can be extremely helpful and save a lot of money. In addition is you get higher performance devices.
You can start adding radios, fans, and TV to improve their quality of life. So now, I'm going to pass it off to Kirsten. And she's going to talk about our six to 12 month prospectus.
KIRSTEN: Great. Thanks, Jackson. So it's important to note that the Lighting Africa project doesn't actually manufacture photovoltaic devices. That is they actually cooperate with small companies which are risk averse, which means at least in the next 12 months, they're interested in the most established manufacturing infrastructure.
For that reason, in the six to 12th month range, we've just looked at silicon. So we've compared mono- and polycrystalline silicon with amorphous silicon. Of course, as you all know, monocrystalline silicon has a higher efficiency but, also, higher price per watt peak.
It's important to note that in the Lighting Africa project because the lifetime of these devices is not limited by the photovoltaic component, but rather by the LED, the CFL, the battery, it opens up an interesting opportunity in that the photovoltaic component doesn't have to last as long as an installation you might put on a roof in Cambridge. It could only, maybe, be three to five years in lifetime.
And for that reason, we could decrease the cost of the encapsulation materials, the commodity materials, the glass, and the backing that would normally be required. So amorphous silicon might be a better opportunity in this application. So let's compare crystalline amorphous for the two consumers that Jackson mentioned in the beginning.
For Consumer 1, who's seeking the lowest cost option, again, we want an integrated module in the solar portable lantern. So for that reason, amorphous silicon doesn't have a high enough efficiency to integrate the device into the SPL. It would require 400 centimeters squared. Because the devices are so small, we're limiting that to the crystalline silicon where, again, you're not going require as many encapsulation materials as you would in a roof insulation in Cambridge. But, still.
And then, for Consumer 2, who's seeking higher performance modules, you could either use amorphous silicon, remove the encapsulation materials, and have a very large area solar cell, which you could, again, bring in and out during the day. Or it opens up another opportunity in that you could use crystalline silicon, encapsulate it with standard glass aluminum frame. And this photovoltaic module could actually exceed the lifetime of the device.
That is a person could buy a solar portable lantern, to which would only last three to five years, and replace the SPL later, replace the LED in the battery but still use the same photovoltaic module. So for both consumers, low cost and high performance seeking consumers, crystalline silicon presents a better solution. With that, I'll pass it off to Ben, who will talk about the one to five year prospectus. So.
BEN: So for the one to five year time frame, we looked at two different technologies, which we've, well, we considered crystalline silicon to offer kind of a baseline for cost. And then, we looked at technologies where we could achieve a cost reduction. We focused on micromorph silicon thin films and organic solar cells.
These are both kind of thin film type technologies. You might wonder why we didn't include cad-tel or CIGS. The reason is because since they contain cadmium, they have to be recycled at the end of their life. And we don't think that was very realistic in this context.
So first, I'll talk about micromorph silicon. And this is a tandem thin film cell with amorphous silicon and microcrystalline silicon. It's on the verge of commercialization within the next few years. Cost estimates are about $0.70 a watt. And efficiency estimates are about 10%.
So first, we considered the low cost option. How inexpensive can we make a basic solar portable lantern? So here, you see the historical cost in 2010. And then, under various scenarios you have the cost estimates for 2015.
The crystalline silicon was assumed to be the same as it is today, basically a dollar a watt. Micromorph silicon was assume to be $0.70. And then, on the far right, we have kind of a really optimistic scenario where we've reduced cost even further by reducing encapsulation materials just for this application. And so we're down to $0.55 a watt.
What you can see is that the costs are going to go down regardless of what PV platform is used. And micromorph silicon provides about a 5% additional cost decrease compared to crystalline silicon. And that doesn't sound like a lot to us perhaps. But it's not insignificant in this context.
Next, we looked at these high-performance options. Because since the cost of the device is dominated by the non-PV materials for these basic devices, as you add more PV material, you leverage the low cost of the PV. So we split us up into three different high-performance products-- the basic, the medium or multifunctional, and the ultra high.
And to kind of give you an idea of what these are capable of, the basic might be able to provide about five hours of light a day and a cellphone charge. The medium one could charge 20 cellphones. So this could act as a community sort of cellphone charging center. And then, the high-performance one could even power things like sewing machines, TVs, things like this, so lifestyle changes or entrepreneurial opportunities. So now, Rachel will talk about organic PV.
RACHEL: OK, so the second low cost alternative to crystalline silicon, that we considered in the one to five year range, was organic photovoltaics. And organics are unique because they're made up entirely of carbon-based plastic material, which means that they're manufacturing costs can actually be substantially lower than that of silicon. And in fact, they're forecast only cost $0.50 a watt by 2015.
And unfortunately, their efficiencies are less promising. Because they're only about 1% to 3%. But Heliatek, which is a Dresden based company, has recently set a new record for almost 10% with their tandem organic cell.
So the first thing we're going to look at is how organic PV could play a role in the inexpensive SPL for the first customer. And this is a plot of the module area required for various power generation capacities. And it compares 20% efficient silicon with 3% and 5% efficient organic PV.
And basically, what this shows is that to generate the same amount of power as a silicon cell, you need a substantially larger area of organics. Because it's so much less efficient than the silicon. So this affects the first customer. Because in order just to generate the 2.5 watts necessary for the SPL, you actually need over 800 square centimeters of 3% efficient organic material, which is not reasonably something that you could fit onto a lantern that you have to carry around with you.
So because the first customer requires a solar panel that could be entirely integrated onto a handheld device, its organic PV doesn't really represent a great option, at least in the one to five year term, because it's efficiencies are so low. OK, so the second thing we're going to look at is how organic PV might be useful to the high-performance modules that are entirely separated from the devices that they charge. This is the second customer.
So this is a plot of the manufacturing cost of the entire device and versus the power generation capacity. And it compares a dollar per watt silicon with $0.50 and $0.35 per watt organic PV. And basically, what this shows-- this is pretty much the same plot that Ben just showed except it compares organics-- is that as we look at a more high-performance device, the cost savings, that you get from switching from silicon to organics, are actually quite substantial.
And in fact, for the same cost as a given silicon device, you can actually get almost twice the power generation capacity by switching to organics. So for this reason, and also because a module area is not really a constraint in this context, because we're just talking about building a module that you're going to leave in your yard, and not carry around with you on a lantern, organic PV is actually an excellent option as a low cost alternative to crystalline silicon in the one to five year range despite its very low efficiency.
OK, so the conclusions for the one to five year term, basically, are that the manufacturing cost of the SPL can be moderately decreased by switching from crystalline silicon to micromorph. And there are greater cost reductions available in the higher performance modules. Because as the lower costs of micromorph and organic PV can be more fully leveraged as we consider a device with greater power generating capacity. So with that, I will give it to Ron for the 10-year.
RON : OK, so moving on to the five to 10 year prospectus, this had a unique challenge in that, oftentimes, research cell modules will have a huge barrier to commercialization. And these are things that the probability of them actually realizing commercialization, in the five to 10 year period, decreases significantly. So what we did is we tried to look at modules that we're not like thermal photovoltaics or interband gap transition cells.
Because we were afraid that the probability of those actually reaching commercialization were very low. So one option that stood out to us was actually an extension of the one to five year period, was the mature organics as the benefits that both Ben and that Rachel mentioned. Specifically, that as the areas of the cell get larger, they could have a very good cost leverage. Because they could power other household items such as TV, fans, and radios.
Granted. also they have extremely attractive dollar per watt peak performances because of their extremely low cost and moderate performances. Also, we expect that commercialization will be set to begin and that, actually, the efficiencies of commercial scale modules will reach 5% to 8%, which are actually pretty good performance modules. Another thing that stood out to us was actually cadmium-free CIGS technology.
And as Ben mentioned, cadmium has a huge environmental impact and would require a recycling process, which is not practical given this context. So we looked at a cadmium-free CIGS technology that would actually have the benefits of thin films in their low cost and moderate performance, but not have the actual environmental concerns associated with them. So currently, in lab, there have been a few options to replace the cadmium sulfide buffer layer.
There's been zinc oxide, and also zinc indium selenide compounds that can, actually, be grown epitaxially in the manufacturing process, making it really easy for roll-to-roll processing. So currently, in the research cell modules, they are reaching about 10% to 12% efficiencies, which is really promising given they're actually, it hasn't been studied for that long. So we expect that in the five to 10 year period, we could actually realize this high-efficiency cadmium-free CIG cells, especially since manufacturing processes have already been developed for the CIGS cells.
So for the long-term conclusions, we think that the cadmium-free CIGS modules be great for Consumer 1 because of their moderate performance and their attractive dollar per watt peak metrics, making them, actually, integratable on a small area, and providing the 2.5 watts for the SPL. For Consumer 2, we actually thought a large, mature organics module in their backyard would be great because of their really low cost, and also because of their ability to possibly charge other household devices as their performance increases. And these are, basically, the conclusions of all my other colleagues in front of me.
The six to 12 month mono- and multicrystalline are the best options. In the one to five year period, micromorph and organics will play a larger role. And in the five to 10 year, organics and CF CIGS stand out as very attractive options. So in conclusion, next generation photovoltaic technologies definitely have the capability, especially as alternatives to current crystalline technology, to provide a green and positive difference in the Lighting Africa project.
[APPLAUSE]
AUDIENCE: Have you worked with anybody, like D-Lab or any other nonprofits that have done this kind of work before? Because, sometimes, it's about the relationships that you can build with the companies, the kind of discounted products that they can give you. And that's, sometimes, kind of takes over.
JACKSON: Yeah, so, I guess, we skipped this slide. Or we didn't get to it. But we actually worked with the World Bank, the Lighting Africa is with the World Bank.
We also worked with people who were working on it at Humboldt State University, and talked a lot to them. And right now, so one thing that's important is that the Lighting Africa project is, they provide a lot of education and information to companies. But the companies are the one that actually build the devices. Does that answer your question?
AUDIENCE: So it's up to the companies to decide which one [INAUDIBLE].
JACKSON: Exactly, yeah. And so the Lighting Africa provides information to them. And our report will be provided to the companies as well. But in the end, it's up to them to decide what's most profitable.
KIRSTEN: And also, there's several companies that the Lighting Africa project works with. And each company provides a slightly different solution. So there's a whole spectrum of the number of watts you have, the number of kilowatt hours. So there are several different solutions based on the different companies.
AUDIENCE: So when [INAUDIBLE] dollar per watt, what about thermal degradation for all [INAUDIBLE] that could be a major problem, right? I mean, like [INAUDIBLE] itself, I mean, that's why they're not, I think, [INAUDIBLE].
RON : So what's, actually, really positive about this project is that we're not really looking for high lifetime devices. So it's, actually, limited by the batteries and by the other components of the SPL. So that's only, they only have about two to three year lifetime.
So we think that even though, obviously, Africa's hot, there the thermal degradation will actually not be the limiting factor in this case, especially if they're encapsulated with relative--
AUDIENCE: So that could increase the cost, right?
RON : No, actually if you reduce, since the cost is dominated by non-PV materials, we could actually reduce the encapsulant costs significantly and still have a relatively good lifetime.
AUDIENCE: So could you comment on that, actually, a little bit? What kind of lifetimes would you expect out of the current production organic cells?
RON : [INAUDIBLE]
JACKSON: Yeah, Rachel.
[LAUGHTER]
RACHEL: OK, great. So, oftentimes, you'll find that current commercialized organic cells only last a couple of days actually. Although it has been proven that certain cells can last up to three years, which would be sufficient for the lifetimes that we're looking at.
And this is also in the one to five year range. We're not talking about doing this tomorrow. So, hopefully, that sort of metric would be improved within that time frame.
AUDIENCE: And you guys have a sense is it an encapsulation challenge or a fundamental materials challenge?
RACHEL: I think it's a fundamental materials challenge. It's the actual--
JACKSON: Yeah, and this actually gives a great place for organics because of our time frame. Usually in the US or, the modules last for 20 or 30 years. But here, the lifetime of the device is really two or three years. So it's a great opportunity for organics.
KIRSTEN: Yeah, and then the opportunity for decreasing module costs. It just doesn't go all the way across the board. It's more important for silicon than organics. Because, again, organics aren't as [INAUDIBLE].
RON : And also I know that hydrolysis of the carbon-based compounds is one of the big problems. So they're really not good for, like, relatively humid environments. And in this case, we're going to be in the sub-Saharan African desert. So it's going, that won't be an issue.
AUDIENCE: Did you, do you have any idea about what market, what the size of the market would be for different possible costs that you outlined on some of those line graphs? Say your total lantern cost is $40 or something. How many people can buy it if that's what the price is?
KIRSTEN: So we've, the top 10% of the population in sub-Saharan Africa earns 40% of the GDP. So there would be very few people in the second customer bracket. Whereas, most people, I guess, earn less than a dollar a day and would be our target for the first archetypal, as the first archetypal customer.
AUDIENCE: So if most of the people earn less than a dollar a day, are they going to save up couple months of income somehow in order to buy a $30 lantern. That's what I'm asking.
JACKSON: Yeah, so currently, the buy back time on these are about eight months. So it's very significant. And that's why there's not widespread proliferation.
But right now, the Lighting Africa project is working on education. And in areas that they've started these educational programs, the proliferation of these products have actually increased dramatically. And people have started saving up for them, and buying them.
And actually, there's one of the main problems with the project that there's a wide scale, there's, like, Chinese companies that come in. And they'll sell very, very cheap devices that are extremely shoddy. They last maybe a few weeks or something.
And they're really cheap. But they don't really do too much good. So increasing education about those, and the Lighting Africa project gives certification of devices that will last a few years. And so increasing education and decreasing buy back time, which is one of the main things we'll increase.
AUDIENCE: Sounds like you do consumer reports.
JACKSON: Yeah.
RON : And also, one of the, I mean, this is actually more towards customer too. But one of the positive things about this project is that communities could pool resources together and actually have their household items be charged together in one person's house, in the middle of the community. Could have that.
AUDIENCE: Yeah, I have a question sort of like all these lights I'm assuming use batteries, like the cadmium batteries or [? lithium ?] batteries, and so on, so forth. And those are hazardous materials. And I'm sure they're either dealing with them or they aren't. So given that fact, doesn't that make your assumption that you ruled out cad-tel or CIGS cells to be incorrect in that you already have hazardous materials in the batteries.
JACKSON: Right. So right now, I think, for the most, they are [INAUDIBLE] in these batteries. Most of the batteries are fairly well sealed and such.
But I think, ethically, we couldn't suggest more hazardous materials. And so you're saying that just because there's already a problem, why don't we just add another one. I don't think that's necessarily a good option. But you're right about the batteries.
AUDIENCE: So for your tier one customer, your lower income customer, you're pretty much only providing them lighting. Is that correct? Or do you think--
JACKSON: Right.
AUDIENCE: --that there's options? I mean, you have a lot of graphs that had area of device in cost, and for the different technologies. Do you have any idea, if I wanted to charge a cellphone, where we'd have to lie on those graphs?
RACHEL: Yeah. It's a good question.
JACKSON: [INAUDIBLE] appendix.
KIRSTEN: Should we skip to the appendix?
RON: Yeah, of course.
BEN: Yeah, so charging a cellphone--
AUDIENCE: I mean, so if you want lighting and cellphone, how much does that add to the cost?
BEN: Yeah.
AUDIENCE: Is there a larger market for that?
BEN: So there could be a very large market for that. Because it doesn't, actually, take that much energy. It takes about four watt-hours to charge a cellphone. So for a very basic device that maybe produces nine watt-hours a day, you could get five hours of light, for instance, depending on the efficiency of your LED, and, then, also charge a cellphone. So it's actually, it's not a great threshold to overcome to be able to charge other things, especially cellphones.
JACKSON: And the non-PV aspect of the cellphone charge is also very cheap.
AUDIENCE: Could you please comment again on why you say that, or do you see higher potential in organics for the one to five year period than in cadmium-free CIGS cells? So why did you get to the conclusion to recommend organics for the one to five and CIGS for the longer term?
RON: We wanted to be conservative. And cadmium-free CIG cells have only been recently not discovered, but researched in the past year or two. So we wanted to be kind of conservative with our estimates and take into account that there might be big hurdles to commercialization. So we put in the five to 10 year period. Thanks.
AUDIENCE: So one of the interesting things about electricity generation in Africa, it seems, is the lack of a legacy system. And so you really do have this question of like, what is the most cost effective way of delivering power today rather than what's the most cost effective way of delivering power when you have electricity grid? But what, do you guys have a sense of sort of the cost per person at which electrification by grid becomes a compelling thing to do?
JACKSON: Yeah, that sounds something we looked into.
[LAUGHTER]
JACKSON: Definitely, I think the cost would be pretty high, at least compared to these devices. I mean, of course, you have a lot of, it's very, very spread out, and a lot of small villages, and what not, which these are targeted towards. One of the other ideas, one of the other concepts that was looked at was a larger scale version of this where you have a large PVA ray or a few panels in the city or the city center.
And then, all the villagers could come in and charge your batteries that way. And they all have little [INAUDIBLE], which was another model that is being looked at.
AUDIENCE: So it sounds like what you're saying is that if you push more towards concentrated populations, then the hereto kind of becomes more favorable where you can have larger installations and central locations that communities pool together and share.
JACKSON: Yeah, that's where we're going.
BEN: Yeah, there's two different demographics. So you have your off-grid suburban. And then, you have off-grid very rural. So often, in the relatively high income bracket, the tier two, these are people living in kind of off-grid suburban areas. There just outskirts of cities and things like that.
JACKSON: And another thing, actually, is that we talk about off-grid a lot. But the actual grid in Africa is so poor that these products are actually very applicable to them. Some people have blackouts daily or weekly, such that these devices become necessary as well.
PROFESSOR: All right, let's thank our speakers again.
[APPLAUSE]
STUDENT 1: So hi, everyone. We are Team 6. And we are going to talk about the potential of simulation softwares to predict the photovoltaic performances. And our team members are [INAUDIBLE], Noami, myself, [INAUDIBLE], and Omar, here.
OK, so this is the figure that you guys are very much familiar with. So we all do know that silicon solar cells show the benefit of high efficiency, whereas the efficiency of the thin film, or emerging photovoltaics, are relatively low. But they are definitely under a spotlight.
Because they do have the advantage over the silicon such as higher absorption efficient, and lower manufacturing costs, and the flexibility. So we are going to simulate based on the materials used in these types of solar cells. So our model, in general, because they do have a lot of benefits, such as it is very much useful.
But it is impossible or impractical to create certain experimental conditions. And also, [? doing ?] assimilation is much more efficient in terms of money and time taken. And also, we can do the fundamental and simple understanding at a very small scale such as atomic scale.
But there's one very critical limitation. That is the accuracy problem. So this means in many cases, the accuracy matters. Like it's not really accurate as the [INAUDIBLE] happens.
So the system to be modeled will be explained in detail by following speaker. However, if I speak, if I say very briefly, it can be divided into three. First, all organic such as P3HT and PCBM system. And the second one is all inorganic, such as lead sulfide quantum dot and titanium [? fullerene. ?]
And the last one is some combination of those, so called hybrid cells. So our aims here, our first, we are going to introduce the two simulation packages and, then, followed by the comparison of the performance by, sorry, performance from the simulation with the values from the literature. And in the detailed study section, we basically change everything in the simulation package, such as layer thickness, temperature, band energy levels including the electron affinity and band gaps, and lastly, the carrier mobility. And then, the presentation will be concluded with sensitivity studies here.
STUDENT 2: So the question raised as to which simulation packages we should use. So you all are familiar with PC1D, which is industry standard for simulating crystalline silicon. However, given that we're simulating organic materials, inorganic materials at very low transport, we opted to go with more generic simulators called AMPS, from Penn State University, and SCAPS. And I'll discuss why.
So with these organic materials, and these thin film materials, traps and recombination are a huge issue. And the question arises as to how to model these traps. So at the highest level of abstraction, that most top-level view, you can just look at the bulk recombination time and simulate with that, which is the approach that's used by PC1D.
The second level of abstraction is to break up your bulk recombination times into the different components. And in particular, Shockley-Read-Hawk recombination is very important for these materials. So as you remember from class, you basically have traps between your conduction band and valence band. You have [? curves ?] that are in these two bands.
And when they recombine, that effectively reduces your current. So both AMPS and SCAPS actually allow for simulating these physical traps. So you can simulate it with-- and these traps have different spectrums with respect to energy.
So for example, you can have traps that decay exponentially from the band edges. Or you can just have discrete level traps that are certain energy level. And you can have traps that have a Gaussian distribution.
Both AMPS and SCAPS allow for that. At the same time, SCAPS allows for spatial non-uniformities for traps. So for example, let's say if I have a layer, and at the top layer, there's a lot of traps.
But by the time, by the bottom of the layer, there aren't that many traps, I can model that in SCAPS. However in AMPS, I only have, I can only model a uniform distribution of traps spatially. So I can't have any Gaussian distributions or anything like that.
The second key component for solar cells are your contacts. For PC1D, you can only feed it shunt resistances and series resistances, which is nice in that it's convenient. But if you don't know them or if you can't measure them because you don't have the cells, then that's a hindering factor.
So therefore, it's nice to be able to model the physics of your contacts by taking the barrier height of your metal and semiconductor, and by looking at the recombination velocity. So both SCAPS and AMPS allows for that. And at the same time, SCAPS also allows for modeling with your shunt resistance and series resistance. So that's nice in that if you have those measurements, you can just do that instead of mucking around with the physics.
So other considerations include the total number of flares that you can simulate. AMPS is actually, has an advantage in that you can simulate up to 30 layers. So if you have graded materials, it's a good approximation to be able to just simulate 30 layers.
They all pretty much do same basic set of simulations, including simulating under dark conditions, under illuminated conditions, and band calculations. SCAPS, and at PC1D also allow for additional measurements, like capacitance measurements as a function of frequency, or as a function of voltage, which is very nice for physics. Other considerations include documentation and ease of use.
AMPS probably had the best documentation. You had an 80-page user manual. SCAPS was this composed of random PowerPoint slides that were thrown on the website. So it's a little bit harder to figure out.
And finally, in terms of ease of use, I'd say PC1D is the easiest just because you don't really delve into the physics. You just type in values. And it simulates.
Whereas, with AMPS and SCAPS, if you get your parameters wrong, it won't simulate, or it'll freeze, or things like that. So here's this brief run through of the user, of the interface for SCAPS. So this is the main page where you set your measurement parameters like your lighting conditions, your voltages.
And then, from there, you can define the structure that you're simulating. So in this case, this is for a p-i-n amorphous silicon structure. And then, you can define the properties for each of those layers. So this, for example, are the properties for the p-type layer.
So these are basic material properties like your electron mobility, your band gaps, so on, so forth. And on the left, you can define your traps. As for AMPS, it's got a little bit more of a minimalistic layout, which is more pleasing to the eye.
So this is like your basic page. And then, from there, if you can define each of your layers. So with that, I'll pass it on to Naomi.
NAOMI: So for six organic materials and six inorganic materials, we found all their physical properties from literature. So we use either well-known properties, or the most common properties for these materials for the most common crystalline structure, or the crystallinity that we would use for solar cells. .
And for some materials, it was very hard to find certain properties, such as the density of states, for example. And we had to approximate these numbers. And then, we used these properties, these basic properties to compare the performance from literature versus the values that we obtained from AMPS and SCAPS.
So for inorganic solar cells, we can see that there's a good correspondence between literature values and both simulation tools. And this is likely due to the fact that inorganic materials have more similar properties to silicon than organic materials. And the simulation tools were based on silicon when they were created.
So we looked at the open-circuit voltage, short-circuit current, the fill factor, and the power conversion efficiency. But then, when we had an organic component to the device, we see that there are more significant discrepancies between literature values and the simulations. So for example, in this case, we had usually an overestimation of the performance with SCAPS and an underestimation with AMPS.
And we also have to note that we're expecting lower performance from our simulations than the literature. Because usually in literature, the values are reported for bulk heterojunctions. So a mixture of the two materials while in the simulation, we use only flat bilayer heterojunctions. So here we can see that we have been very little fill factors for SCAPS and very high efficiencies. So that's the main discrepancy.
And for organic solar cells, we also obtained a very high efficiencies from SCAP as well as open-circuit voltage, and some very significant underestimations from AMPS, of the short-circuit current, and the efficiency. But due to the [INAUDIBLE] use of SCAP, AMPS was taking longer to run. And it was more sensitive to small changes in property.
Sometimes we would have convergence failures. So we decided to look mostly at SCAPS. And we varied some parameter properties to look at how these softwares would respond to changes in the physical properties [INAUDIBLE].
STUDENT 4: So this is a where the detailed study comes. And the first thing we have looked at is the temperature [INAUDIBLE]. So only a representative figure is shown here.
So as you learned in the class, if we increase the temperature from 250 to 350, the efficiency and open-circuit voltage is the equation. And this is kind of expected, because decreasing. So we can explain the reduction of open-circuit voltage by, yeah, so if we increase the temperature, the electrons gets easier to excite to the conduction band. So that effective band gap is actually decreasing.
And also we didn't show the short-circuit current data here. But there's a slight increase of short-circuit current. And this could be, also, explained by the same thing, like it's easier to get excited to the conduction band.
And the second thing we have looked at is layer thickness. And also, here, there only a representative figure is showing here. So if we increase the thickness from 0 to 1,000 for the [INAUDIBLE], the efficiency and the short-circuit current is initially going up.
And after certain point, the efficiency, [INAUDIBLE], is slowly going down. And this is also expected. Because for the observing material, when we first initially increased the layer thickness, it gets more, initially more light.
But after certain point, absorbing more light effect is already saturated. So increased layer thickness just means the more distance to the electrodes. However, whereas for the acceptor material, there's no significant of effect of the layer thickness on the performances.
AUDIENCE: It's like will you help me with this graph, which ones are--
STUDENT 1: Question?
AUDIENCE: Are the dotted lines the current?
STUDENT 1: Yeah, so the solid line is efficiency. And the dashed line is current.
AUDIENCE: And one is for SCAPS and one is for AMPS?
STUDENT 4: No, All the simulations done in SCAPS actually.
STUDENT 5: So I'm going to talk about how you change your particle size, you will change your band gap, and also change your result of the simulation. So as you know, changing your particle size of the [INAUDIBLE] will change the band gap of the material. And this is very important for organic solar cell.
From the table above, you can see when we change the lead sulfide, lead sulfide particle size from bulk material to 2.4 nanometer, you can change your band gap from 0.4 increase to 2.1. So we will use these numbers into the simulation tools to simulate the efficiency of two system.
One is lead sulfide zinc oxide. The other one is lead sulfide titanium. So you can see the white dot on the figures. That's mean efficiency. The highest efficiency occur at the band gap is around 1.5.
So that's mean the particle size is smaller than the bulk material. So which just mean if you use smaller particle size with the higher band gap, you can get a higher efficiency because two reasons. The first is your [INAUDIBLE] increased because your band gap increased.
And the second is that your [? fill ?] factor also increased in both systems. So how is this simulation [INAUDIBLE] of this simulation compared to the literature? So we choose the lead sulfide titanium system.
So we used the structure in the right, above figures. Now, there's titanium lead sulfide. And then, the [INAUDIBLE] is gold.
And then, the world record efficiency, right now, is around 5.1%. So the particle size of this literature used is five nanometer. So find the nanometer in our simulation. The result is around 4% to 5%, which is, it's very close to each other.
But our simulation result can help the future direction of this research regime. Because you can see the simulation result. If you change the particle size to the lower, to the smaller particle, you can increase the efficiency to 8%.
So that's how simulation can helps the research. So I would just pass to the sensitivity part.
STUDENT 6: We also to determine the critical parameters to which [INAUDIBLE] that the performance [INAUDIBLE] we calculated sensitivity. Sensitivities are calculated from changes in efficiency, [INAUDIBLE] by varying parameters, simulation parameters, or material properties.
They were expressed in terms of change, a 1% change of efficiency in the parameter of relative to basis value. So as we can say, we found four major parameters. And the [INAUDIBLE] two things out, there were no band gaps and electron affinity, which are well-known material properties. And others are [INAUDIBLE] thickness and temperature, which can be controlled the [? web ?] by it's [INAUDIBLE].
So from this sensitivity [INAUDIBLE], we strength, I mean, confidence of our simulations.
NAOMI: So to conclude, after doing all these simulations, we found that the these simulation softwares were useful tools to simulate efficiency trends as opposed to absolute values of the performance parameters, which remain challenging to simulate, especially for organic compounds. However, the sensitivity analysis was good to validate that the most well-known material properties seem to have the highest influence on the efficiency. And we can usually also control temperature and layer thickness, which we're also very, very influent for the efficiency.
So this project was useful to collect a lot of material properties. And we could potentially create a database of properties that could be useful for solar research. And this could be included in such, in a project such as the Materials Project, which incorporates lots of databases about materials for different applications.
And then, if you could connect these material databases to the simulation tools directly, it would be good for a user who simply wants to input a pair of materials. And then, we could get the output as performance parameters directly. And finally, we want to thank Professor Buonassisi, as well as the research groups from Gent University and from Pennsylvania State University for the use of their simulations softwares.
[APPLAUSE]
AUDIENCE: You said you had a problem modeling the bulk heterojunction organics? I mean, because it's bulk heterojunction instead of a bilayer. Did you try just modeling a single layer with the properties that are a mixture of materials?
NAOMI: So.
STUDENT 1: Yeah, so actually, we tried to do bulk heterojunction modeling as well. But it is limited by the simulation capabilities. So we can define the layer. Yeah, so you can define several layers in here. But we--
STUDENT 4: We can show the software to them.
STUDENT 1: Uh-huh. So if we want to do the bulk heterojunction, we could do, just put one layer where the effected material properties. However, the hard thing was, the shifts at the band gap, actually, the parameter. However, you could not define the band gap.
Because even if we did just one layer, it is a mixture of two different layers. So it's hard to define band gaps. So it will mess up everything. So we couldn't.
AUDIENCE: Your last slide made me think of some recent publicity from a group, Harvard, I think. Some guy trying to use the distributed computing idea similar Folding@home or [? Study at Home, ?] wants people to download his screensaver, and screen thousands of different organic materials, I think, for photovoltaics.
NAOMI: Yeah.
AUDIENCE: Do You know what software they used for that?
NAOMI: Don't know.
AUDIENCE: Custom software.
AUDIENCE: Custom software? OK. So can you guys go back to the, you had an array of bar charts, at some point, when you were comparing the different simulation tools.
Free Downloads
Video
- iTunes U (MP4 - 285MB)
- Internet Archive (MP4 - 285MB)
Subtitle
- English - US (SRT)