Flash and JavaScript are required for this feature.
Download the video from iTunes U or the Internet Archive.
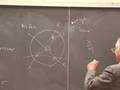
3D Symmetries, Point Groups...
PROFESSOR: To mention, most everyone did extremely well on the quiz. But I sense that there's still some of you who have not yet come to terms with crystallographic directions and planes, and you feel a little bit awkward in distinguishing brackets around the HKL and parentheses around HKL. And there are some people who generally get that straightened out, but when I said point group, suddenly pictures of lattices with fourfold axes and twofold axes adorning them came in, and that isn't involved in a point group at also.
Again, a point group the symmetry about point. A space group is symmetry spread out through all of space and infinite numbers. So let me say a little bit about resources.
I don't know whether you've been following what we've been doing in the notes from Buerger's book that I passed out. That was hard to do is because we did the plane groups, and he doesn't touch them at all. So now we're back following once again Buerger's treatment quite closely. So read the book. And if you like, I can tell you with the end of each lecture, this stuff is on pages 57 through 62.
The other thing. As you'll notice, this nonintrusive gentleman in the back is making videotapes of all the lectures. These are eventually going to go up on the website as OpenCourseWare. We were just speaking about that, and it takes a while before they get up, but I have a disk of every lecture. So if there's something you didn't follow or a place where I chewed my lines and you want to go back over it again-- not that that happens very often-- you are more than welcome to ask me to borrow and borrow the disk if you want to review it. So that's another resource.
And then I will regularly throughout the term give hard-copy handouts of some of the things that we're doing, particularly when it involves geometry. And when we move on to three-dimensional geometry, unless of the graphics is really tight and precise, it's hard to follow what's going on.
So in that vein, one of the first things I wanted to pass out-- the only other one for today-- is a demonstration that in fact in the Group 23 all you need is a single twofold axis oriented along the normal to a face and a single threefold axis coming out of one body diagonal. And that gives you all of the axes you are going to get into 23.
So when this comes around, there are number of steps that you can perform letting the axes work on each other. And if you start with just the single twofold axis and the single threefold axis-- which the symbol suggests is all you need, there's only one kind of each-- if you look at a cube along its body diagonal, the twofold axis that's coming out of one face gets rotated into directions normal to all the other faces if you rotate by 120 degrees. So the little diagram in the upper right hand corner of the sheet hopefully convinces you of this.
Now we've got three mutually orthogonal twofold axes and one threefold axis coming out of a body diagonal. So the vertical twofold axis swings that around by 180 degrees to give you another threefold axis along a body diagonal. And I labeled that one 3 prime in the middle diagram at right.
And then if you take the axis 3 prime and repeat it by the second of the twofold axes that we have along face normals, that in the middle diagram on the right hand edge takes 3 prime and repeats it to 3 double prime. Then finally, the third twofold axis that we generated repeats the threefold prime axis to the remaining threefold axis along the fourth body diagonal.
So the results start with one twofold axis oriented along the normal to a cube and one threefold axis along the diagonal of the cube, you get axes coming out of all faces and all diagonal. And there's a staple on that sheet for reasons that I don't understand, but it there was, probably on the surface of the Xerox machine when I went over there.
So let me now return to our next step, and that is to add what in the language of group theory is called an extender, a new symmetry operation that can be added to a preexisting group that will generate new operations. And let's see what sort of theorems we need to describe what we should look for and in which particular orientation.
We've got these 11 axial combinations, and these are frameworks that we can hang mirror planes on. So let's look at a first simple combination. Suppose we have a twofold axis, and the only nontrivial operation there is a rotation through 180 degrees. So this an axis A pi.
And again, the ground rules are that if we're to add a mirror plane to this axis, which along with identity constitutes a group, we can't create any new axes. So there are two ways we can do this. One is the three-dimensional analog of something that we have already done, namely to pass a mirror plane through the twofold axis. And this is the group that we found as a two-dimensional Point Group, and we called it 2mm.
And to do that, we used the theorem that says that if you take a rotation operation A alpha and combine it with a reflection operation that goes through it, you get a new reflection plane, sigma prime, that's at an angle alpha over 2 to the first. So what gave us the second mirror line in two dimensions, in three dimensions that would give us another mirror plane that's at right angles to the first. And this will give us a three-dimensional symmetry, which is also called 2mm.
So it's nothing more than taking the two- dimensional Point Group and letting it come out at the board at you-- Man, that'll give you nightmares when these things are coming out of the paper at you-- and that is a valid group 2mm.
There's another way that we can add a mirror plane to a rotation axis though which will not create any new axes, and that's to add the mirror plane-- the reflection operation sigma I should say since it is a combinations of operations-- exactly perpendicular to the locus of the rotation operation. And that reflection operation sigma then just flips the rotation axis end to end. And the rotation operation just swirls the locus of the reflection plane around within its own locus and doesn't create any new reflection plane.
So in the particular combination A pi, if we add a mirror plane perpendicular to that as the operation sigma, this would be then all of the operations of a twofold axis over all of the operations of a mirror plane. So we've got now a combination of a twofold axis with a mirror plane. We've got the same thing here.
To distinguish these two combinations, we'll write this as a fraction 2/m. And that literally in words is the way we've added the twofold axis. It is sitting over the mirror plane and gets reflected down into its other end when the mirror plane act on. So it's just language. It's nice though, as we said some weeks ago, if our language have some descriptive content to so when we look at it we can remind ourselves of what it means. So two 2mm means the mirror planes are parallel to the axis that contain it. 2/m means the twofold axis is over the mirror plane. It goes through and pierces it.
What I would like to ask though is have we got a group yet? And let's take a first object-- let's call it right handed-- rotate it by 180 degrees. You get a second one, which will stay right handed. And then repeat it by a reflection operation in the mirror plane, and we'll get a third operation. Reflection changes chirality, so the third one is left handed.
So we are performing the sequence of operations A pi followed by sigma. And let me append just so we make no mistake and not confuse it with 2mm that the reflection operation is normal to the mirror plane.
So question, what is the net effect? And lo and behold, we have stumbled over-- if we had not been clever enough to invented it and suggested it early on-- the only way you can get from 1 to 3 in one shot is to turn it inside out and change its chirality by projecting it through a point which is the location where the twofold rotation pierces the mirror plane.
And what this is going to do is to change the sense of all three coordinates. This is going to take the coordinates XYZ of object number 1 and change them into minus x, minus y, minus c. And what we're doing is inverting the motif, turning it inside out as it were, into an enantiomorph. And we have discovered as soon as we combine a pi with a perpendicular reflection plane, a new operation which we'll call in words inversion. And the symbol that used to describe this is a one with a bar over it, and we'll see why later on. But this is a onefold axis with an inversion center sitting on it, and that's what an inversion center by itself is.
The implication is that they're going to be other axes that we can abbreviate such as 3-bar, 4-bar, and so on. So this is analogous to a situation that will come later where we really need a new notation.
So we've fell headlong over a new type of symmetry operation, and we should consider taking inversion and adding that to the rotation axes by themselves as an extender. Obviously, if we take inversion and add it to a mirror plane, we're going to get A pi. If we take a twofold rotation, add it perpendicular to a mirror plane, we get inversion. If we put inversion and put it on a 180-degree rotation, we'll get the mirror plane back. So these things all permute one to another.
You may even remember some time ago we asked in general terms when do two operations permute without changing anything. And the answer is if these operations to leave the locus of the other one alone. And the mirror plane obviously leaves the locus of the rotation operation unchanged. The rotation operation spins the mirror plane around in its own plane and doesn't create a new axis. And the inversion center leaves the mirror plane alone and takes the twofold axis top to bottom.
So those three operations, inversion A pi, sigma are the three nontrivial operations that exist in the space. The fourth one is the identity operation. So here is the set of elements in the group that we will call 2/m. 2/m implies three operations inversion, a 180-degree rotation, reflection in a plane perpendicular to the axis, and the identity operation.
And I'll leave it to yourself for you to convince yourself that I can rotate and then reflect or I can reflect and then invert. And all of these operations do not create any new motifs in the set. The group multiplication table, in other words, contains just the four elements, 1, 1-bar, A pi, and sigma.
So the full pattern that corresponds to 2/m consists of four objects. It'll be a fourth one down here. The twofold axis tells you have this fellow is related to this one. Inversion tells you how this one is related to this one. And the mirror plane tells you how this one, number 1, is related to number 4. And the identity operation tells you how 1 is related to itself.
So, again, as we've seen in the set of operations that constitute a group, there's a one-to-one correspondence between the transformations that are elements of the group and the number of objects in the pattern.
So we've made one combination, and what we found from this is a new transformation inversion that involves changing the sign of all the coordinates in a space through a point which is called the inversion center. Questions?
If not, let me quickly rattle off other Point Groups in this family. We could take the operation A pi/2 in 90-degree rotation and add this perpendicular to mirror plane.
Let me now say something that I've said again many times before. The pattern of objects that will result is the pattern of objects that's produced by the initial group-- let's say a fourfold axis-- repeated by the extender. And the operation sigma perpendicular is the extender.
So the pattern, without making any big deal about it, is going to look like this square of objects reflected down below the mirror plane. So it'll be one going down like this. One like this. One like this. The operation A pi sits perpendicular to the operation of reflection, so there will be an inversion center that arises at the point of intersection. And indeed the square above can be inverted through this point-- little hasty repairs there-- and every one up above gets inverted down to an enantiomorph below.
So all of these guys up on top are right handed. All these guys down below are left handed. So this is another group. This is 4/m in international notation, a fourfold axis perpendicular to a mirror plane.
There is also, unfortunately, a Schoenflies notation. The international notation tells you what you've got, a twofold axis or a fourfold axis perpendicular to a mirror plane. The Schoenflies notation tells you how you derived it. And the symbol for a twofold axis is C2, so this is a twofold axis. And what we did was to add an extender consisting of a horizontal mirror plane. Schoenflies calls this one C2h; Group C2, which is a twofold axis; a horizontal m is the extender.
So Schoenflies tells you how you make it. The international notation tells you what you get as a result. Schoenflies notation here would be C4, that's the symbol for a fourfold axis, and the extender is an h.
And then without making any big fuss about it, if I do the same with a sixfold axis, I will have six objects related by a sixfold rotation axis. If I take that sixfold axis and put a mirror plane perpendicular to it, these will be reflected down to a hexagon of enantiomorph equidistant below the mirror plane.
That's not terribly good, but it's not terribly bad either. So this would be called 6/m, Schoenflies notation C6h. And the operation A pi exists in a sixfold axis, so there is an inversion center but also arises as a new symmetry element at the point of intersection.
So with reckless abandon, you can continue on here and derive noncrystallographic groups for all the even-fold axes and derive an 8/m and a 12/m and 16/m. Lovely symmetries. I wouldn't want to draw them, but they're still symmetries. They all have an inversion center in them, but they're noncrystallographic. So we don't have to worry about them for prism purposes.
I left one out because it introduces a complication that is kind of curious and interesting. Any questions on what we've done here? Yes?
AUDIENCE: The 2mm, it's just the same-- Schoenflies notation is just C2--
PROFESSOR: Schoenflies notation for three dimensions is exactly the same as in two. So the three-dimensional version where this extends in a direction that is normal to the two-dimensional space of our two dimensions.
Two dimensions it was this. Now just imagine them coming out of the blackboard at you. The symbol for this one was 2mm. The symbol for this one is also 2mm, the same thing. The mirror plane is a vertical mirror plane. So the Schoenflies notation is exactly the same as what we used per two dimensions. It's called C2v. We've added a vertical mirror plane.
And again, horizontal and vertical. Horizontal is horizontal with respect to the axis of higher symmetry. Vertical is vertical with respect to the two-dimensional space of the two-dimensional Point Group, parallel to the axis in three dimensions. So that's the vertical indication.
So let's, though, tuck that away for future reference. We've got two kinds of extenders. We've got a horizontal mirror plane, and we've got a vertical mirror plane, and these are extenders that we should consider adding.
So we've taken care of 2/m, 4/m and 6/m. 2mm, 3m, 4mm, and 6mm are just the extensions into a third dimension of what we've seen and come to love in the two-dimensional space.
Let me now turn to the threefold axis. And this is a curious one. Threefold axes require fewer symbols to indicate the vertical mirror planes because there's only one independent one. But let's see what would happen.
And now I'm not going to attempt to draw these in three dimensions anymore. I'm going to use a stereographic projection. And what I'll do is use a solid dot for a point that's up above the equatorial plane and an open dot for one that's down below the equatorial plane.
So my stereographic projection of 4mm would look like. This is the fourfold axis. This is the mirror plane. And I've got one up that gets reproduced by the axis to give me a set of four. All of these are, let's say, right handed. And then directly below them is another set of four repeated by reflection, and these are all left handed.
And then there's an inversion center at the point of intersection. And I'll indicate that by the little open circle sitting right on the fourfold axis. So there is a projection of what 4/m looks like.
So let me now do the same thing for a threefold axis. And I'll add to the triangle of points that a threefold axis would generate. So these guys are all of one chirality. Let's say right handed. Then I'll reflect them down, and I'll get three objects that are down. And that's what 3/m looks like.
Is there an inversion center here? No. No, because the operation of A pi is missing. And it was the horizontal mirror plane combined with A pi that gave us the inversion center with all of the even rotation axis.
So one of the things we have to say here is that there is no 1-bar that's present, which means in this instance, unlike the other ones, we have another option. So we can use the operation of inversion as an extender too. So we're going to get another group out of the threefold axis besides this one. And this one we will name 3/m or C3h in Schoenflies notation.
They're six objects here, so there should be six operations in the group. So let me number these guys up on top as number 1, number 2, number 3. And 1 is related to itself by inversion. There's an operation A 2 pi/3. And that tells me how the one that's up is related to the second one that's up and how the left-handed one that's down is related to the one that's directly below number 2.
There is an operation A 4 pi/3. And that's the same as saying 8 minus 2 pi/3. And that tells us how the things that are separated by 240 degrees are related, both up and down.
I know how 3 up is related to 3 down and how 1 up is related to 1 down and 2 up is related to 2 down. This is all with the horizontal mirror plane, which I'll call sigma h. That is a total of one, two, three, four-- whoops-- one, two, three four operations. I need six.
Let's ask how is this one that's up related to this one that's down? I just got rotation operations and reflection. The only way I can get from this one number 1 to this one number 3 left that's down is to take two steps to do it. I can't get from this one up here to this one down here unless I rotate 60 degrees and then invert.
If I move over to 3-bar. This is A 2 pi/3 with 1-bar as an extender. The pattern would, again, look like what are threefold axis does. But then if I repeat this set of three by inversion, the two triangles above and below are skewed. The ones down below are enantiomorphs. The three that are up are of opposite chirality. And this is a new type of pattern.
And in international notation, what do we call this? It's a threefold axis. But how do we indicate a symbol for three with inversion sitting on it? Let's ask if we know how each of these objects is related to each of the other. So here's 1, 2, and 3; 1 goes to 2 by A 2 pi/3; 1 goes to 3 by A minus 2 pi/3.
Let's put some numbers on here for the ones down below. Let's call them 4, 5, and 6; 1 goes to 6 by an version. How do I get from 1 up to 4 that's down? I can do that only by taking two steps. Rotate 1 from here to number 2. Don't yet put it down. First invert it.
So 1 to 4 involves the operation A 2 pi/3 followed immediately by inversion. And I go from 1 up to 5 down by doing the operation A minus 2 pi/3 followed immediately by inversion. And then 1 goes to 1 and itself by the identity operation. So I have six objects, one, two, three, four, five, six operations. Yes?
AUDIENCE: In the cases where you're rotating and inverting, does it matter which way to the other?
PROFESSOR: No, it shouldn't because they leave each other alone. So I can rotate from here to here and invert. Or I can invert from here to here and then rotate. It's the same transformation. Again, they permute if the two loci of the two operations leave the other locus alone.
Maybe the enormity of what we've shown here has not sunk in. This is a new two-step operation. We can't describe it any simpler than saying, rotate and not put it down yet, follow up by inversion. Yes, sir?
AUDIENCE: Couldn't we express that in another way by sort of extending the three-directional glide plane by saying invert, then transform by some vector that's parallel to the glide plane?
PROFESSOR: Maybe they do in space group, but as soon as we introduce a glide plane, you've got an operation that's half a lattice translation. And that means you've got to have a lattice translation and double the lattice translation, so--
AUDIENCE: Oh, we don't have to worry about that.
PROFESSOR: --when we're in a space group, yeah. That could be present. But not for a point group because the ground rules are at least one point has to remain immutably fixed in space.
So this is a two-step operation, and what we're going to call it is rotoinversion. It consists of as a first step an operation by rotating alpha from 0.1 to a virtual point number 2. But before you put it down, you will invert it to a new object number 2 which is of opposite chirality.
So here then are the operation of the group that results when you combine a threefold rotation axis and add to it an version center as an extended; A 2 pi/3; A minus 2 pi/3; a rotoinversion operation through 2 pi/3 and then inverting; a rotoinversion operation of A minus 2 pi/3 followed by inversion. And the symbol that is used to represent that new two-step operation is putting a bar over the top of the symbol for the axis. And then, finally, we have inversion by itself. So that's a group rank 6.
The Schoenflies notation is called C3i because we got this group by adding an inversion to C3, the threefold axis. The international notation picks up on putting a bar over an axis to indicate a rotoinversion operation. So this is called 3-bar in the international notation. So there is a new group, and it is an oddball. It sort of stands alone from the other groups of the form C3h.
This we derived by using the rotation of the threefold axis and adding 1-bar as an extender. So there's no mirror plane in this.
AUDIENCE: That's not the same as--
PROFESSOR: That's the same as 3/m, no. 3/m is C3h. 3-bar is C3i, different extender added to the same subgroup 3C.
AUDIENCE: What was the definition of 3/m?
PROFESSOR: Oh, we never really finished that. That if we need the six operations that control the group, we'll have a sixfold rotoinversion axis. But this pattern looks just like the triangle produced by 3, and we add an reflection operation as an inversion, and the 3 go down.
So if we ask how every one of the top is related to one underneath, that's by this horizontal mirror plane. If I want to know how I get from this one that's up to this one that's down, then I've got to rotate through 60 degrees and invert. So that would be a rotoinversion operation.
Let us to extend this idea of a rotoinversion operation. And we would find this eventually in adding different extenders and falling headlong over this rotoinversion operation as we did here with 3-bar. But let me in this case start by defining a 4-bar operation.
And this would contain the operation A pi/2 followed immediately by inversion. And we'll call this step A pi/2-bar. So let's try to do that and see what we get.
Start with a first point, number 1. And that's up, so it's a solid dot. And let's say it's right handed. If we combine that with a rotation of 90 degrees. Not yet put it down. That's a virtual motif. Before putting it down, we inverted it. We would get one that's down, and it would be left handed. Do the operation again. I'll put the little tadpole inside. Do the operation again. Rotate 90 degrees and invert. We're back up again.
So this was 1. This is 2. This was 3. And that's up. Do the operation again. Rotate and invert. And here is number 4, and it's down. Do it a fifth time, and we're back to where we started. So this is a crazy pattern. It's a pair of objects that's up and a pair of objects that's down. So there's a twofold axis in there. That twofold axis A pi leave the pattern invariant.
But there is no way of specifying the relation between the two that are up and the two that are down other than doing this two-step process of rotating 90 degrees and then inverting. So there is actually in this pattern a new type of operation analogous to 3-bar, and it's called a 4-bar axis. And it's indicated geometrically by drawing a square because there's a 90-degree angular symmetry to this. But a twofold axis inscribed inside of it because this is a pattern that has a twofold symmetry.
So something that has this symmetry is the symmetry of a tetrahedron. And if we draw a line from the upper edge to the lower edge, this is the locus of a 4-bar axis.
International notation this is called 4-bar. That's how we generated the pattern. The Schoenflies notation is an S, little bit of exotica. This geometric solid is something that's called a sphenoid. And sphenoid. Is the Greek word for axe. And you can imagine a handle put onto this thing, and it does look kind of like an axe. You could splits firewood with a thing like that. It looks like a tetrahedron, but in a tetrahedron, it's either elongated along the 4-bar axis are squished. It doesn't have to be regular. So this is called S4, and the S stands for sphenoid.
AUDIENCE: Is it part of a regular tetrahedron?
PROFESSOR: No, no. A regular tetrahedron would be something where all of the edges had equal length. And what we're doing is taking one edge and the edge that's opposite it and either stretching it or squishing it.
So there are two edges. This one, and this one, which are the same length. And then these four inclined edges have a different length. It could be either elongated or squished. But it's not a regular tetrahedron.
If those three distances were equal, then geometrically it would be a tetrahedron. But strictly speaking, a tetrahedron is not a tetrahedron, just as a square prism with eight sides approximately equal can't claim to be a cube unless there's symmetry present that demands that this be true.
In this case, the 4-bar requires that these four edges inclined to the 4-bar axis be of one length. And this have to have the same length. But there's nothing that constrains all six to the edges to be of identical length. So it's not a tetrahedron, so squished or deformed tetrahedron.
So there is another two-step symmetry element that we would not have been clever enough to think of had we not discovered this sort of rotoinversion operation when we added inversion to a threefold axis. A 3-bar axis is a step that's present when you add inversion to a threefold axis. So 3-bar, what we call it for short, is identical to a threefold axis plus inversion sitting on it.
A 4-bar is not equal to a fourfold axis with inversion added to it. A 4-bar is something that you cannot describe any more simply than saying there is a two-step operation in there, and it's a group of rank 4.
Let me finish by setting up the task of going through this systematically. We have 11 axial combinations 1, 2, 3, 4, 6, 222, 32, 422, 622, 23, and 432. So there are 11 of those.
We want to examine as extenders a vertical mirror plane that would be one extender. We should add that to each of these symmetries. We already done a lot of these. We've done pretty much up here.
We could add a horizontal mirror plane. Or we've encountered inversion when we added a mirror plane perpendicular to an even-fold axis. We could add inversion as an extender. And to be complete, we should add to our list of axes in quotation marks, the 4-bar axis, having discovered it.
And does that do it? Is there anything else we could do to these axes that would leave them invariant? .
AUDIENCE: What about 2-bar?
PROFESSOR: 2-bar; 2-bar would be rotate 180 degrees and invert. So 2-bar is identical to a horizontal mirror plane. So that's nothing new. We're already running a little over time so-- Yeah?
AUDIENCE: 3-bar?
PROFESSOR: 3-bar is 3 plus 1, and we call at 3i, so this one down here. This is 3-bar. We describe it for short as that, but it really is a threefold axis with an inversion center sitting on it.
This thing is distinct because it's not a fourfold axis with inversion sitting on it. A 4-bar is a 4-bar is a 4-bar. You can't decompose it as a twofold axis is a subgroup. That's only half the story.
I don't want to keep you anxious, not anxious to find out what the answer is but anxious to get on your way and go home. So let me submit that when we have more than one rotation axis, such as 222 or as in 32, if we put the mirror plane in normal to the principal axis, we'll call that a horizontal mirror plane.
If we add the mirror plane through the principal axis, we could pass it through the threefold axis and the twofold axis, pass it through the vertical twofold axis and the horizontal twofold axis. We will call this a vertical mirror plane. And that's all we could say for a single axis, the mirror plane was perpendicular to the axis or passed through it.
But when there's more than one axis, another thing we could do would be to snake the mirror plane in between the twofold axis. In that case, this twofold axis gets reflected into this one. But I haven't created any new axes. So that is going to leave the results of Euler's construction unchanged.
I can't similarly put a vertical mirror plane through this first twofold axis but in between the other two. In that case, this is no longer 222 because these two mirror planes are equivalent by reflection, so I want to drop that at very least.
So in any case, without belaboring the point, it's late. I could do for each of the groups that involved more than one axis I could add a diagonal mirror plane, or I should try to add a diagonal mirror planes. And this means interleaved between axes that are present in these combinations, added such that no new axis is created. But the addition clearly is going to be a new disposition of symmetry elements arranged in any different fashion and space.
So the game's afoot. This is what remains to be done next. What I'll do for next time is prepare a chart that looks like this that has the results of all of the unique combinations shown and then hand out pictures of stereographic projections of all the results. I think once you know how to play the game to go through and do every single one in detail is probably not necessary. If you know how to do some of them and you know all the tricks for adding extenders, you could do it if you had to.
All right. So, again, sorry we started late and sorry that we last long as well.
Free Downloads
Video
- Internet Archive (MP4 - 201MB)
Audio
- iTunes U (MP3 - 11MB)
- Internet Archive (MP3 - 11MB)
Subtitle
- English - US (SRT)