This resource is available as audio only.
Flash and JavaScript are required for this feature.
Download the track from iTunes U or the Internet Archive.
Description: This lecture covers innate behaviors and motivation along with the approach of Konrad Lorenz to studying animal behavior and the fundamentals of ethology.
Instructor: Gerald E. Schneider
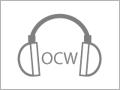
Lec8: Innate behaviors, mot...
The following content is provided under a Creative Commons license. Your support will help MIT OpenCourseWare continue to offer high quality educational resources for free. To make a donation or view additional materials from hundreds of MIT courses, visit MIT OpenCourseWare at ocw.mit.edu
PROFESSOR: Have a little bit to finish up from last time. After last Wednesday's class, I had another job to do, and I totally forgot about this class until yesterday. And I realize-- thanks to the TAs-- that I hadn't posted the homework properly. It was actually in the slides, which I also hadn't posted.
So I got all that done and delayed the due date until Wednesday. It's not a very hard homework, so you should have no problem with it. But that means Wednesday, because you've got that homework to do, I won't give a quiz.
The reading this week is a little more difficult than what you've been doing. It's all posted online. A book by Konrad Lorenz-- The Foundations of Ethology book. It's still some of the best material on ethology. Of course, there's been recent work, and we will be looking at some of that online, as you already have.
Now let's just finish talking about biological rhythms. We got started with that last time. We saw this measure of a hamster-- just one of a number of measures of hamster locomotor activity. And you can see this would be true whether you were studying rats or mice.
But hamsters are particularly good for this kind of work, because their circadian rhythm is synchronized to the light/dark cycle-- pretty much exclusively by the light/dark cycle and nothing else. I've kept them in constant lighting conditions. And even though I was feeding them regularly at the same time every day, and there were noises in the hallway outside the lab, pretty much followed the usual human rhythm of activity of a workday. And yet they weren't affected by those things. It was only the light that influenced their circadian rhythm.
So this is the free-running rhythm here. That's where they started constant light in here. Even with the optic tract cut, they still responded to the light.
The lights were going off here. And you can see they began to get active before the lights go out. Hamsters are what you call a twilight animal. They become active when the shadows are long-- especially in the evening. And they go out and forage for food and then come back to their tunnels. And then they enter there.
So that's when they become active. They stay active at least for several hours in the early part of the dark period. And then you can see they become very inactive. So we mentioned this proof of the endogenous clock mechanism. These free-running rhythms are good evidence for it. But the brain studies led to the discovery of a little cell group in the hypothalamus.
What led to the discovery was quite simple. The anatomical tracing methods got better. They hadn't noticed with the earlier tracing methods-- including the earlier nodal methods-- they often missed this particular projection to the hypothalamus. And this suprachiasmatic-- this nucleus we abbreviate SCN-- suprachiasmatic-- right above the optic chiasm and the crossing of the fibers coming from the retina. And in lateral-eyed animals, most of them cross to the other side.
And other people used other methods. They weren't all using mammals, of course. They were using fruit flies and many other animals. And these genes were initially discovered in the fruit fly. The period gene critical for the endogenous clock, but the clock gene also plays important roles.
And this is a side view of a hamster brain stem where the hemisphere's removed. This shows the main optic tract. And here's where the fibers come in. The eyes would be out here. And so it's connecting with the chiasm here. And that little nucleus sits right there. This just shows what it looks like on [INAUDIBLE] stain.
And here's a fiber-tracing study from some of my work with a postdoc. You see the fibers terminating. These are all coming from one eye, but they terminate equally on the two sides of the brain. It's not a specifically cross projection. Probably the most primitive of the retinal projections.
And here you see what they look like on your higher magnification, where you see the particularly sensitive method of tracing allows us to see the individual axons. If we looked at much higher magnification, you can see individual terminals and individual axons and terminals.
And if this is a ventral view of a human brain with the eyes pushed to the side there, there's the optic chiasm. This is actually a number they've stuck on there for instructive purposes. But that's the optic chiasm, and the nucleus sits right above that.
So why do animals sleep. Why do we sleep? You can answer. As you know, we talked about "why questions." We first talked about Tinbergen's four questions-- different ways of answering that question, "Why?" Here we're asking a behavioral question-- why do we sleep? Why do animals sleep? They all sleep.
First of all, there are adaptive pressures that made the evolution of the sleep instinct occur in all animals. It's a fixed-action pattern. And animals, in fact, show specific activities associated with preparing for sleep, going to sleep. They make nests-- some of them make nests every night. Some of them simply go to their nest. They engage in certain little preparatory activities-- humans do this, too-- and go to sleep. So we can talk about the adaptive pressures, which we'll do first.
And this instinct depends, of course, on brain mechanisms. And they've discovered special roles of not only the suprachiasmatic nucleus in determining the rhythm of activity-- which affects the time we go to sleep-- but changes in the brain. Talk about the neuromodulator systems-- systems of very widely projecting axons, where the activity of one little cell group can make widespread changes in brain activity. There's a number of such systems in the brain-- probably at least seven. We talk mainly about just a few of them.
So first of all, adaptive pressures. The body does need rest and recuperation. That is undoubtedly true, and there's evidence for that-- deterioration in bodily functions that can happen when you get inadequate sleep. Need to conserve energy stores. If we were using energy all the time, we would run out. That would vary of course with the species of animal and the availability of food.
Need to avoid predators. Animals tend to be active in either the daylight or nighttime-- day-active and night-active animals. We're day-active animals-- except at MIT, where we tend to be night-active. But this is talking about natural behavior in a sort of the primitive state the way humans evolved.
And we can evolve mechanism to avoid certain kinds of predators. It's difficult to evolve mechanisms to avoid all kinds of predictors. So most animals, by being day-active, need a place to sleep at night to avoid the night predators.
And the night-active animals often became night-active. We think mammals evolved as nocturnal animals initially. All the evidence indicates that. It was reduction in their vision and increase in audition and olfaction. They became active at night on the forest floor avoiding the day-active dominant species-- the dinosaur group-- in particular the reptiles, which generally used vision to hunt.
Another is in development. You may be aware that, early in development, babies spend a very large amount of time sleeping. They wake up to feed. Initially that's about all they're awake for. And then they sleep.
And their brains are busy when they're sleeping. In fact, it may be a particular role of dream sleep. They spend a lot of their time in dream sleep. What are they dreaming about? They haven't experienced much, yet. Well, they have many built-in mechanisms, and they're not being used to guide behavior. The baby can't even do much, can't move all that much yet. But that doesn't mean the brain mechanisms aren't engaged. And that is one possible role of the dream sleep.
And then, finally, discovered in more recent years-- there's a lot of experimental psychological work on this-- and the role of especially deep sleep period in memory consolidation.
And I expand that a little bit, because it's undoubtedly true that we don't just consolidate new memories. We edit old ones, because the environment changes, and it would be maladaptive to maintain the memory for things that are no longer there in our current environment. So at least for those things we have to change the memories. We have to edit them. And that happens also during sleep.
So then the sleep instinct we will call a fixed-action pattern. It's triggered by the endogenous clock, which is then trained by the light/dark cycle. And then the action of neuromodulator systems. And as I mentioned, these have widespread connections. The best-known ones are the ones using serotonin, norepinephrine, and acetylcholine. All of these systems have very widespread connections in the brain, and there are others in addition that have been discovered.
And one thing that's not been investigated so much-- whether it's actually more difficult to study, but it certainly could be studied a lot more, and that is that there are substances secreted into the cerebral spinal fluid at the onset of sleep. And if you move some cerebral spinal fluid from a sleeping animal into an awake animal of the same species, you can make the awake animal get sleepy, too. And this has been known for some time, but it's been very little studied.
But that concerns mechanism, which isn't our main concern here. I want to say a little bit more about the behavior-- things you may or may not know about in sleep schedules. Many humans, especially before electric lights, sleep biphasically. There's a first sleep and a second sleep. And they get up in between, and they're active for awhile. It's most common when daylight is less than 10 hours a day.
And it has these names. If they're English-speaking, they will talk about first sleep and second sleep. And of course we know that in many hot countries a siesta is very common. They go to eat lunch, and the stores all close. They don't open again until at least 2:00 in the afternoon. So between 12:00 and 2:00, if you're a tourist, you might wander around and not find anything's open. Of course the tourist industry is adapted to Westerners not practicing the siesta. So they do keep more things open. But siestas are still very common in many countries. That's another kind of polyphasic sleep.
It's very common in animals. So for example, if you take hamsters, when they enter their inactive period it doesn't just mean they go to sleep. They do go to sleep for awhile, but they're not sleeping the entire inactive period. They periodically get up. They do things in their nest area, in their tunnels. But they stay below ground. They don't come out and forage.
It's when they enter their active period-- that's their foraging time. Now of course, hamsters wouldn't have to come out all the time-- especially, say, a hamster with babies might not have to forage for food as long as there's a few males around with piles of food. They can steal from them, because they horde food. But some species don't horde as much. Actually, mice horde, rats horde, but not to the extent that animals are specialized for it like hamsters with pouches that can hold a lot of food that they collect and bring back to their burrows.
So what about animals that live in areas like the Arctic or Antarctic where there's continuous light or darkness for parts of the year? And there's been some studies of this.
There was a study published in 2005 on the circadian organization in reindeer. They studied two different groups. They found that they abandoned the daily rhythms of activity in the summer where there's continuous light-- both groups do. In at least one of the groups, they abandon this activity cycle in the winter when it's continuously dark. So they're not always following this hamster-like or human-like activity rhythm.
Goldfish have been even more complex. Sometimes we like to use these organisms, like we like to think of them as simpler species. But in this case it turned out to be much more complex. Their activity in many of them is higher during the daytime, and they feed more in the nighttime.
That is, their activity rhythm and their feeding rhythm can be out of sync with each other. And some of them, though, are just the opposite. They feed in daytime and they might sleep at nighttime. It's not so predictable with goldfish, and it hasn't been studied enough to know what are the adaptive reasons why they're shifting their activity patterns like this.
And they've looked at free-running rhythms in the goldfish-- and they do have rhythms, but often the feeding rhythm and activity rhythms are not in sync with each other even when they're free running.
So there have been various studies of total sleep need in humans, and military organizations and businesses have been very interested in this. And they've tried the experiments to increase efficiency of sleep-- increase efficiency-- of course, activities. And they get many different kinds of results. And the only thing that's really certain is that individuals do vary a lot.
I had one textbook I used when I was teaching the 901 class, that he insisted that we never need more than six hour's sleep. He said that everything above six hours is just pure pleasure.
But when you look at the actual research, there's not support for that. Some people definitely seem to need more sleep. If I ask you how much you sleep, it would probably average between eight and nine hours, and then you'd say, well, I need that, but I don't get it. Well, there are consequences. What's one of the things that happens if you fall too far below your sleep needs? Your immune system is affected, and you're more likely to get sick. So that is a serious thing to think about.
I thought I was a night person for a long time, and then I found out I just needed more sleep. And I could get up and study perfectly well in the morning. In fact, I did a lot better job than late at night trying to stay awake and study.
All right. Everybody does have the biological clock. They've not found humans that do not have this rhythm of sleep and waking. They have looked at the effects of these genes.
For example, studied deletions of the clock gene in mice. Very interesting-- it causes very abnormal regulation of feeding and metabolism. If they're homozygous mutants, they become hyperphagic and obese just like they had some brain damage. And even though the brain damage that causes that doesn't seem to directly affect that gene, you get very high leptin levels in the blood, abnormal fat storage in liver cells. And you get some of these abnormalities even in the heterozygous animals.
So now I wanted to talk about the Lorenz readings. I've given you three assignments. This will be about the first reading. And then on Wednesday and Friday-- Friday, actually, I've given you readings for Friday and Monday together, because the readings are longer and there's many more questions I want to deal with. So there'll be four classes looking at this Lorenz book. And then there will certainly be more ethology as the class goes on. We'll come back to Scott and we'll do some other readings. We'll come back to Tinbergen, also.
The reading is from a section of the book in which he talks about fixed motor patterns. But he comes from a period where they used fixed-action pattern and fixed-motor pattern almost synonymously, and then they began to realize-- and he makes this very clear in the book, too-- that the meanings are somewhat different. So I'm calling it fixed-action pattern, because most of that chapter that you're reading, he's dealing with the more general term, "fixed-action pattern."
Now when he does make it very specific that this has different components, the concept that even though it embraces these different meanings, they weren't separated. And it took them a while to realize what the separate components were. So what are they? If we deal with the separate components of a fixed-action pattern, which is the general term we use for an inherited pattern of movement.
You have the actual motor pattern-- and that's called the fixed-motor pattern. And that's the most unchanging part. It was studying fixed-motor patterns in doves and pigeons and in waterfowl where Heinroth and Whitman made the discovery that these movement patterns are just like physical characteristics of the body. They can be used to even find homologies across species. You find it's the same in all members of a species, and so forth.
They were looking at courtship patterns of these birds. Courtship patterns it's particularly clear, because when the fixed-motor patterns are performed, they're almost always performed in the very same way. That's not true of many fixed-action patterns, as we will see.
So what else? There's a fixed-motor pattern. What else is there? Well, the motivational state. I made it clear before-- there has to be underlying motivational state where the motivation is high enough so the result of the motor pattern actually reduces the motivation. The animal is motivated to do that action.
But we also have the stimulus, say. It doesn't just happen. It happens with a stimulus. So we talk about innate recognition. Animals do inherit-- innately they have the recognition of certain stimulus characteristics. They respond to other members of the species by certain characteristics of the species. In fact, innately they don't recognize individuals at all. All they need is these key stimuli.
So here in this where I'm outlining this slide, I talk about the motivation or drive first. They have the drive to search for a certain stimulus situation. And this drive, in the Lorenz theory, is called an action-specific potential. It's the drive level-- the motivational level-- that builds up in the brain of the animal that increases the likelihood. The higher it gets, the more likely he is to show the fixed-motor pattern.
So then the innate recognition. And here we talk about the innate releasing mechanism. So that is actually referring to a mechanism in the nervous system and sensory system. And we connect parts of the brain. There's innate recognition. The brain is tuned. Because of the inheritance of the animal, the brain is wired in a specific way, and he responds to a specific stimuli. And then, finally, the fixed-motor pattern.
So now we've said a little bit about reflexes several times. We've talked about fixed-action patterns. Let's try to draw simple information flow models. And this is the way I've drawn them. So I show a reflex there at the top. I put the sensory side in green, the motor side in blue. And then you don't have the motivational side for a reflex, so there's nothing in red. I put that in red.
So the reflex is simply a pathway from stimulus to response. It goes through, you could say, the sensory analysis. We can make the response specific to certain characteristics of the stimulus.
And then on the motor side you have to activate in a pool of neurons that end with a motor neuron. Motor neuron has the fiber going right up to the muscle. And notice I'm showing other kinds of inputs that can influence it. These could change with motivational state, too. Certain reflexes might become more likely in certain states of motivation. But generally this can fluctuate a lot, these other inputs. But the main input is always the triggers. The actual response is just one.
But for the fixed-action pattern you can have different stimuli that can trigger it. And there can be a series. We call them the key stimuli-- the inheritance stimuli. Now I'm not saying they're always fixed. I'm not talking yet about how these things are shaped by learning. I'm just talking about what's innate-- a series of stimuli that trigger the innate-releasing mechanisms.
That affects the level of the action-specific potential which also has endogenous inputs, shown by the red arrows. This is why this builds up over time. It has durability. The action-specific potential goes up and it doesn't just go back down unless this is actually discharged.
Then it connects to the motor system in a very interesting way, because many fixed-motor patterns are actually a series of actions. The lowest threshold is usually search behavior. The right stimulus isn't there for eliciting all of these components of the fixed-motor pattern. So the only thing you get is search.
And then, at the higher thresholds, the thresholds change for eliciting the various components. It's often a chain of movements that are all part of the same fixed-action pattern.
All right. So that's a simple way to characterize it. So to learn more about this, it's interesting to go through some of the other Lorenz concepts. He talks about intention movements. So what are intention movements? You should be able to think of examples from animal behavior and from human behavior.
If your level of hunger-- the action-specific potential of that particular pattern of that particular effect-- the fixed-action pattern of eating-- is high, you will show intention movements when there's actually not even any food around. And if there is food around, in fact you will show intention movements before you start to eat, because you'll be affected by the timing by other people around you you're going to eat with and so forth. What is an intention movement here?
Well, people lick their lips. They salivate. They do various things that give away their intention to eat.
What do you do when you're motivated to leave this room, and I'm starting to go over time a little bit? I see all kinds of intention movements out there. I see you packing your bags, I see you shifting in your seat. I mean, these are all intention movements. They're anticipatory movements that in most fixed-action patterns occur.
An animal like a goose, when he intends to fly, always goes through these series of moments. Cranes his neck and emits a call. He shakes his bill. Then he will start to unfurl his feathers. But that's only the third stage. Stage five is when he actually leaps into the air. He goes through the series of movements every time. Well, the initial movements can be considered intention movements.
And it's interesting, because they can evolve on their own, so to speak, because they can serve important social signals. just think of the intention movements to mate. Animals make these intention movements before they mate-- preparatory movements. But those can evolve, too. So they serve as signals to other members of the species.
And that was clear in the studies of even back early in the 20th century, when Heinroth and Whitman were studying fixed-action patterns in geese and pigeons and doves. Some of the things they were looking at had in fact evolved as signals that were closely related to the actual fixed-action pattern that they evolved from. But they become separate. They become signals important in social life.
And that's particularly obvious when we deal with aggressive behavior. And many aggressive encounters in animals are ritualized. It doesn't mean they're actually going to attack, but the threat movements they make that usually don't lead to an actual attack are signals to the other animals of the aggressive intent of the animal. And so studies of aggressive behavior have been particularly helpful in understanding this evolution of signaling functions.
And a lot of courtship patterns which have become quite elaborate in many species-- particularly birds-- are like this. They're elaborate methods of communicating intention to mate. In fact, during that whole time at any time the male is displaying his intention to mate via the courtship dances that he's doing, the female can change her mind and fly away. But if she doesn't change her mind, then, of course, eventually it will lead to mating.
And remember, we talked about jackdaws and kittiwakes. We talked about the kittiwakes, how much of their aggressive behavior does not lead to actual attacks, but they showed a lot of ritualized aggressive behavior. And you see that in jackdaws as well. It's rare when they actually attack. And when they do, the whole colony responds. It becomes confused. And pretty much everything gets toned down without much damage being done.
So if a lot of these fixed-action patterns are actually not like these simple courtship movements that always are executed about the same way, but they actually are a series of moments-- how do you know you're dealing with the same fixed-action pattern if it's a series of different movements? Lorenz goes through an elaborate discussion of this, because people that are just getting into ethology and studying animal behavior find this very confusing, and it takes a long time in studying an animal when you learn which behaviors belong to a specific sequence and will lead to a certain fixed-action or fixed-motor pattern.
The first one he points out is predictability-- that someone that's studying the behavior finds that one little behavior always leads to another. So a good ethologist, if he's experienced, he can reliably predict the next movement in a series. That was true of Seitz's studies in the cichlid fighting behavior of the fighting fish. It was true of Lorenz's studies of geese and of ducks, and it's true of the studies of doves and pigeons by Whitman.
And when the intensity of the motivation increases, the behavior shifts. But it never shifts. They tend to go through certain stages. Like I mentioned, there's five stages in the flight take-off by a goose. He never jumps from stage one directly to stage five. You can tell where he is in the sequence. And in fact if he's not really going to fly, he'll never reach stage five.
But wait a minute. Can't the animal just switch to another motivational state? And maybe that's all he's doing when you see a different movement. Well, animals do switch motivations. But think of an animal that's motivated to attack and he's motivated to feed. Can he switch suddenly from one to the other? Generally not.
Think of an angry human that's also hungry. Does he switch suddenly and sit down and eat? No. It takes them a while to calm down. You don't you shift your motivational state so rapidly. But if you're dealing with a sequence of different movements that all have the same drive behind them, then you do switch. But just not from stage one to stage five, but stage one to stage two, stage two to stage three, and so forth. Or you might drop down one level if the motivation is decreasing.
I point out here that first of all the same stimulus that elicits all the different behaviors in a sequence-- these are the key stimuli that increase the motivation to do a certain behavior. It's the same stimulus that elicits all the patterns, whereas different stimuli are involved if it's a different fixed-action pattern.
So Number 5 here is just similar. It's spelling out details of this readiness to perform that's fluctuating as a single thing determining what patterns you're actually seeing. That unity that ethologists observe, though, isn't always so apparent in baby animals. It does have to develop in many of them. Experience can be very important in the way these things develop.
Now this is a difficult concept, but let me try to go through it anyway, because Seitz was very important in working out some of the principles of ethological investigation and the principles of ethology that Lorenz was talking about. He was an ethologist who, together with Lorenz and a number of others, were working on these things we're talking about.
And he had this method he called the method of dual qualification. It was used to assess how effective a dummy stimulus is. How do you determine how effective a stimulus is when the animals you're studying have all different levels of motivation? It's not an easy thing, because a highly motivated animal could respond to a dummy stimulus that's really not the most effective dummy-- not the most effective stimulus pattern. But an animal less motivated might not respond much at all to that. A very highly motivated animal, he will respond to the less-effective stimulus. Well, how do you know that the difference between two stimuli isn't just due to having animals with different motivational level?
So Seitz realized that what he had to do was expose the animal to the dummy stimulus first, and then, immediately afterwards, what happens if he executes the motor pattern? His motivational level goes down. Every time the animal executes-- the fixed-action, fixed-motor pattern-- becomes less motivated to do it. So show him the dummy stimulus, see how he responds, record the response, and now expose him to the most effective stimulus, which is usually, if you're studying aggressive behavior, another male of the same species in full fighting colors. And see what response you get there.
Well, if the dummy stimulus had been very effective, he would respond much less to that most effective stimulus. If it weren't very effective, he will respond much more. And by looking at the difference between those two, he could judge much better the effectiveness of the dummy stimulus. So that's why you've got to realize there's two variables here-- the action-specific potential level-- the level of drive, which decreases every time he sort of forms the movement, and then varying effectiveness of those stimuli represented by the dummy stimulus.
So this is just what I went over. It's not easy, of course, to apply, but it does increase the ability to judge dummy stimuli. And one of the things that Seitz discovered in doing all this is something about the stimuli. Stimuli usually consisted of a number of key stimuli. It wasn't usually just one thing, but various properties of the stimulus were effective in eliciting the stimulus. But what we discovered was that each key stimulus sort of summated with the others.
So you could show it in a flow diagram like this. The innate releasing mechanism was triggered, so the drive increased and the likelihood of the response increased. If these were key stimuli, their effect summated. Two are better than one. All three is better than two, and so forth. And often stimulus three was just as effective as stimulus one. But the two together, their effects summated.
And he called that the law of heterogeneous summation, because the stimuli could seem very different with each other, but they still, as far as this response, they simply summated in their effectiveness.
All right. So the effectiveness of stimuli, though, can change over time. We already know that when you elicit a fixed-action pattern, the animal-- you've reduced his level of motivation. Now he's a little less likely now. And some things he's a lot less likely. Some he's a little less likely. That varies with what fixed-action pattern you're dealing with.
So they coined the term "action-specific fatigue" to talk about this decrease in motivation. It seemed like it was a fatigue. It was not a very good term. And the problem with thinking about this, or interpreting the behavior, was what about stimulus-side changes they were habituating to?
So this guy [? Precktal ?] actually studied this a lot, and he showed you could get separate effects on the motor side and the stimulus side-- or he called it the receptor side. He was studying gaping reactions in birds.
This is what I just said-- that action-specific fatigue is not such a good term, because it looks like fatigue, but it's not really fatigue at all. It's just less motivation.
Let's talk about an animal I study so much-- the Syrian hamster-- and talk about, say, the orienting movements he makes. Now the hamster is always motivated to get sunflower seeds. I've not been there, but suddenly I show up, and I show him the sunflower seeds, and he makes an orienting movement. But I don't give it to him.
If I keep showing him the seed in the same part of the visual field, he habituates. He will respond a few times, and then he stops. He's habituated. If I show him another part of the field, he would be more likely to respond. And eventually he's habituated.
It recovers over time. If I just wait awhile, I can get them to respond again. Now sure if I reward him, then he's going to keep responding. I'm just talking about what he does when the stimulus is novel, and what he does when the stimulus becomes less novel. He'll keep responding only if he's rewarded.
So let's take another behavior. But this is even more dramatic, because there's no real reward except that he's still alive. It's anti-predator behavior. Say I've built an artificial living situation where he lives in an underground tunnel. But he has to come out to get water or food. And so I know when he's going to come out, because I know his rhythm of behavior. He'll come out about the time the lights are going off. I have them going out fairly rapidly, so I know more precisely when he's going to come out.
So he comes out. He's very cautious. He's very afraid of predators. I have it so that he has to cross an open area. So here comes the hamster. And he's cautiously hugging the ground, moving across the open area towards the food and water I have in the opposite corner of this field area.
Now I make a noise. I take a piece of paper and crush it. Crunch! The animal runs. You think hamsters can't move fast, but in this situation they move very, very fast. And they run back to the tunnel. In fact they run down the tunnel-- sometimes all the way to their nest.
So I'm patient. I wait. And the animal eventually, of course-- usually fairly soon, because he's got to forage for food and water-- he comes up again, and he starts the whole process again. I let him get out in the middle of the field, crush the paper again, and he runs back to the tunnel. But this time he doesn't go very far in the tunnel, and he turns around, and he comes back a lot sooner.
If I do it a third time, he runs over towards the tunnel entrance and stops. He doesn't even go in the tunnel. If I do it a fourth time, he stops and pricks up his ears. So what's happening? He's habituating to that noise. So the next time he comes out, now if I use that same stimulus, he will continue on and get his food or water.
But if I change the sound-- say I jingle some keys or I take my fingernails and scratch a piece of wood-- it can be fairly soft sound, but the hamster can hear it very, very well-- I get the full-blown response again-- running back to the tunnel just from the novel. And again, I can selectively habituate the animal to one sound or another.
Let's say he's responding to this novel sound. If in the very next time I use the crushing paper again, he retains his habituation. It's very specific. He's learned specifically which sounds are novel, which are not novel. It's only the novel sounds that he's afraid of, because they could mean a predator.
Now what he's doing when he freezes or runs is he's looking for predators. In fact, when he comes back from his tunnel, his head pops out of the tunnel. He doesn't just run out. He spends a lot of time scanning for predators on the horizon and above.
So that's selective stimulus habituation. And it depends on novelty. So the stimulus becomes less and less effective with repetitions. And it's affected by the modality. We'll come back to this next time. I'll start by talking about what Lorenz calls tool activities-- the multi-purpose action patterns.
Free Downloads
Audio
- iTunes U (MP3 - 17MB)
- Internet Archive (MP3 - 17MB)
Subtitle
- English - US (SRT)